the Creative Commons Attribution 4.0 License.
the Creative Commons Attribution 4.0 License.
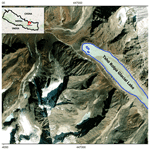
Glacial lake outburst flood (GLOF) modeling of Tsho Rolpa glacial lake, Nepal
Rijan Bhakta Kayastha
Sunwi Maskey
In recent decades, the Tsho Rolpa glacial lake has witnessed a significant increase in its surface area. Situated in the Eastern Himalayas at an elevation of 4552 m a.s.l. (meters above sea level), this lake has drawn attention due to the potential risks of glacial lake outburst floods (GLOFs). To comprehensively study and prepare for future GLOF events from Tsho Rolpa, advanced modeling techniques were employed, utilizing the National Weather Service BREACH (NWS-BREACH) and Hydrologic Engineering Center's River Analysis System (HEC-RAS) models. The assessment included the evaluation of dam breach scenarios, especially 20 and 40 m breaches, using the NWS-BREACH model to estimate breach hydrographs. This analysis revealed peak flow rates ranging from 8198 to 26 662 m3 s−1 for the respective breaching scenarios. Additionally, considering a change in the lake area over 20 to 40 years, the peak flow ranged from 8226 to 10 662 m3 s−1 for the 20 m breach scenario. The peak flow estimates obtained from NWS-BREACH were subsequently integrated into the HEC-RAS model to simulate peak discharge and flood heights at varying distances downstream of the lake outlet. To understand the consequences of a GLOF, the impact on downstream areas was assessed through flood inundation maps, and precautionary measures are recommended.
- Article
(4721 KB) - Full-text XML
- BibTeX
- EndNote
Glaciers are highly sensitive to climate change due to their relatively quick response to temperature variation: climate cooling results in glacier advance, whereas warming leads to glacier retreat. Therefore, glaciers are excellent indicators of climate change, which has recently had a significant impact in high-mountain glacial environments. Hence, recent glacial retreats and concomitant glacial lake formation/expansion in mountain areas serve as an example and infallible evidence of climate change. Glacial wastage or recession, most often of debris-covered glaciers, causes climate warming due to exposed low-albedo surface area, and the resulting formation of glacial lakes and their expansions are intertwined. In other words, glacial wastage initially creates an environment conducive to glacial lake formation and enlargement. In the later stages of glacial wastage, supraglacial and proglacial lakes create conditions that can enhance and accelerate glacier recession. Sometimes, this leads to potent terminus calving into the lake, which is even reported to be a climate-independent glacier ice-loss mechanism, thereby making the glacier terminus increasingly unstable. This process of glacier terminus ice calving into the glacial lake can, in turn, trigger a glacial lake outburst flood (GLOF), among other glacial lake failure mechanisms such as suddenly high discharge from a seepage point, the collapse of a moraine by a huge ice and snow avalanche into the lake, or an earthquake. In light of this situation, understanding the response of glaciers and glacial lakes to rising air temperatures has become an essential aspect of water resource management as well as the management of GLOF disasters.
In 2010, there were 5701 glacial lakes in the Third Pole region, which includes the Pamir–Hindu Kush–Karakoram–Himalayan system and the Tibetan Plateau (Zhang et al., 2015). Landsat 8 Operational Land Imager (OLI) data from 2014 revealed more than 8200 glacial lakes and an overall lake area of more than 830 km2 (Chen et al., 2017). These glaciers are melting rapidly, leading to the formation of new glacial lakes and the expansion of existing moraine-dammed lakes. Some debris-covered glaciers have supraglacial lakes at their termini that are surrounded and dammed by the moraine formed during the Little Ice Age. Those glacial lakes emerged during recent decades and are now also expanding, mainly by calving at their termini. Understanding the behavior of glaciers and glacial lakes is a vital aspect of GLOF disaster management. Moreover, tracking glacial lake evolution and the study of the morphology around them is important for estimating GLOF danger from the lakes. Mitigation and prevention of GLOF hazards are urgent issues that need to be addressed in the context of water resource development and conservation in the Himalayas. However, the recession of glaciers and the concomitant formation/expansion of glacial lakes may not be synchronous in the entire region. Differences in many influencing factors, such as the climatic variability, local topographic effects, and thickness and spatial distribution of debris cover, lead to differences in the size, number, distribution, and type of glacial lakes as well as disparity in the recessional behavior of glaciers (e.g., faster/slower retreat or even the existence of advancing glaciers). Within the Hindu Kush–Himalayas region, the eastern part contains the highest number of glacial lakes; the lakes in this area are larger in size and the majority are proglacial in nature. In contrast, in the western part of the region, most lakes are supraglacial, less numerous, and smaller. In Nepal, the eastern part also has more and bigger glacial lakes. Tsho Rolpa is the biggest glacial lake in Nepal. The main objective of this study is to estimate the discharge and inundation scenarios downstream if a GLOF occurred under present or future (40 years from 2021) conditions.
Tsho Rolpa glacial lake is located in the Rolwaling Valley, Dolakha District, in the central Nepal Himalayas (27°52′ N, 86°28′ E; 4546 m a.s.l., meters above sea level) and forms the headwaters of Rolwaling Khola, a tributary of the Tama Koshi River (Fig. 1). The lake had been rapidly expanding on the terminal part of the debris-covered Trakarding Glacier from at least 1958 (surface area 0.23 km2), when the Survey of India performed a topographic survey, to the late 1990s (surface area 1.55 km2 in 1999), when mitigation work was carried out (Chikita et al., 1999; ICIMOD, 2011). The lake has been considered to be the most potentially dangerous glacial lake in Nepal, especially before engineering work was carried out in 2000 to lower the lake water level and reduce the level of risk with respect to a possible GLOF (ICIMOD, 2011).
The flood hydrograph is an important input parameter in GLOF modeling. The other input parameters required are the digital elevation model (DEM), the surface area of the glacial lake, the maximum depth of the lake, and the volume of water in the lake. Furthermore, different characteristics of the dam, such as the top and bottom elevation of the dam, the width of the dam's crest, the dam's maximum height, and the slopes of the dam's faces play an important role in determining the flow hydraulics over the dam. The material properties of the dam affect the rate of structural erosion; therefore, these will also be taken as input parameters (Westoby et al., 2014). The ALOS PALSAR DEM has been used to create a terrain model to set up the modeling and inundation mapping.
3.1 GLOF modeling
The GLOF modeling process includes breaching the moraine dam, its maximum discharge at the time of breaching, the discharge and water level at different locations downstream, and the creation of a flood inundation map for the downstream area. Risk analysis can be done after this information has been established. Table 1 shows the input parameters used in the Tsho Rolpa GLOF modeling. The research design is the same as that shown in Fig. 2 of Maskey et al. (2020). The Froehlich (1995) model is used to estimate the maximum discharge at the time of dam breaching, the National Weather Service BREACH (NWS-BREACH) model is used to breach the dam, and the Hydrologic Engineering Center's River Analysis System (HEC-RAS) 2-D model is used to create the flood inundation map for the downstream area.
3.2 The NWS-BREACH and HEC-RAS models
The NWS-BREACH model has been used to predict the breach characteristics (size, shape, and time of formation) and the breach outflow hydrograph in this study. The estimated peak discharge from the NWS-BREACH model is verified with the Froehlich model (Froehlich, 1995). Very similar values were found; hence, the peak discharge from the NWS-BREACH model is adopted in this study. It was assumed that the flow reaches its peak discharge following a linear rise before decreasing over the same period of time. The parametric model uses empirical observations of previous dam failures, such as the breach width–depth relation, the time of breach formation, and the breach depth, to develop the outflow hydrograph.
Table 1Input parameters used in Tsho Rolpa GLOF modeling.
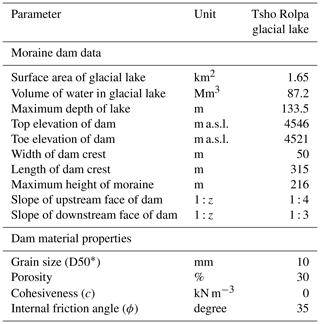
* A total of 50 % of grains have an average size smaller than 10 mm and 50 % are bigger.
However, observations of such parameters are not existent for moraine dams, rendering the parametric approach infeasible. In this regard, the breach erosion model has some advantages over the parametric breach model, as the critical properties used by the model are measurable or can be estimated within a reasonable range from a qualitative description of dam materials.
The HEC-RAS 2-D model is used in modeling GLOF inundation. The details of the NWS-BREACH and HEC-RAS models are adopted from Maskey et al. (2020) in this study.
Based on available data for the Tsho Rolpa glacial lake from 1959 to 2021 (from ICIMOD until 2011 and from Google Earth satellite images thereafter until 2021), its projected area in 2040 and 2050 is shown in Fig. 2. A linear expansion of the lake area is considered. With these areas in 2040 and 2050, the volume of water in the lake is estimated, and GLOF modeling is carried out. The area of the lake was 0.23 and 1.65 km2 in 1959 and 2021, respectively, based on previous reports. Using linear expansion, the area of the lake will be 2.33 km2 in 30 years and 2.53 km2 in 40 years.
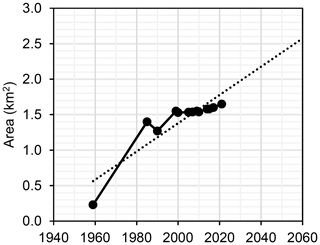
Figure 2Variation in the area of the Tsho Rolpa glacial lake from 1959 to 2021 and beyond. The dotted line shows a trend line.
The possible breaching time and peak floods are estimated for breaching scenarios of 20 and 40 m using the NWS-BREACH model. The estimated peak discharges are 8198 m3 s−1 in 4 h 10 min and 26 662 m3 s−1 in 4 h 30 min for the 20 and 40 m breaching scenarios, respectively, as shown in Fig. 3. Similarly, the maximum estimated flood heights are 11.4 m at 20 km downstream of the lake and 14.7 m at 185 km downstream of the lake for the 20 and 40 m breaching scenarios, respectively, as shown in Fig. 4. The peak discharge due to the 20 m breach was comparable with the GLOF modeling of ICIMOD (2011), in which the peak discharge was estimated to be 7242 m3 s−1; this is a reasonable value with respect to the lower surface area and volume of water in 2009 compared with that in 2021. However, the timing of peak discharge in the present study is a little too high, possibly due to the high volume of water at present. Moreover, the flood height is lower than the aforementioned previous study, possibly due to changes in the river morphology at present. The peak flood height due to a 40 m moraine breach at around 185 km downstream of the lake is mainly due to the river cross section.
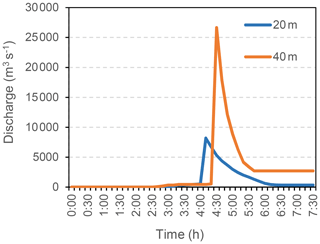
Figure 3Variation in the peak discharge from the NWS-BREACH model for a respective 20 and 40 m breach in the Tsho Rolpa glacial lake moraine.
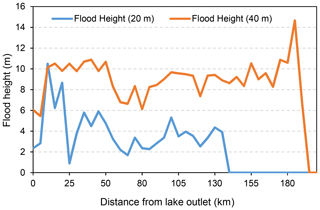
Figure 4Distribution of the flood water height for a respective 20 and 40 m breach in the Tsho Rolpa glacial lake moraine.
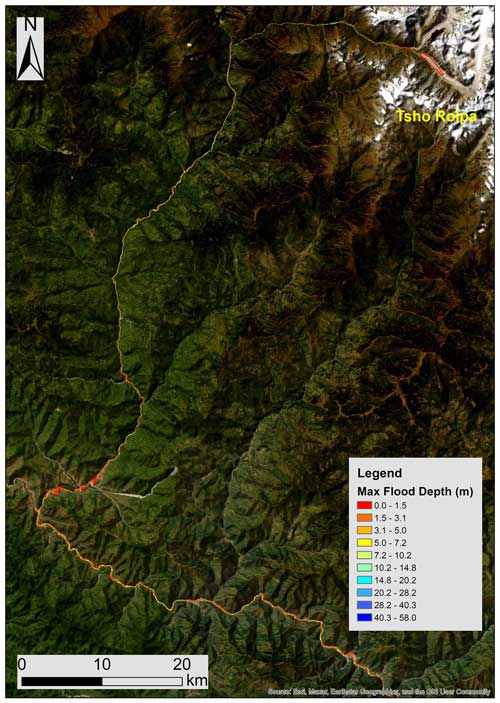
Figure 5Inundation map of Tsho Rolpa GLOF for a 20 m breach in the 2021 lake scenario. Maximum flood depth is shown in the legend.
The inundation map due to a Tsho Rolpa GLOF for a 20 m breach in the 2021 lake scenario generated using HEC-RAS is shown in Fig. 5. The maximum flood height is only 1.5 m. The NWS-BREACH modeling is also carried out to estimate the peak discharges for a 20 m breach in 20, 30, and 40 years (from 2021) to check the future GLOF scenarios. The estimated peak discharge for a 20 m breach scenario in 40 years is 10 662 m3 s−1, as shown in Fig. 6. The estimated peak discharge in 40 years from the 2021 scenario is increased by 2464 m3 s−1, which seems to be reasonable compared to its 0.88 km2 area increase.
GLOF modeling of the Tsho Rolpa glacial lake has been carried out successfully and has provided estimates of the peak discharges in the 2021 lake scenario and for 20, 30, and 40 years from 2021. In the extreme case, in which a 40 m lake outlet is breached, the estimated peak discharge is 26 662 m3 s−1 185 km downstream in Sunkoshi River, Udayapur District. This point is about 25 km downstream of the confluence of the Dudh Koshi River in Sunkoshi; hence, a GLOF in the Dudh Koshi River basin can cause a compounding effect if an outburst flood occurs at the same time. At that time, the estimated maximum flood water height is 14.66 m in the Sunkoshi River. Although a GLOF due to the 20 and 40 m breaching scenarios showed a sufficient lead time for early warning and human evacuations, mainly due to a long stretch of the river, there might be ponding during water transit due to possible landslides or debris carried by the flood water. Therefore, precautionary measures, such as community-based GLOF early-warning systems and mechanisms allowing close observation in the case of GLOF events, should be established in GLOF-prone regions.
The ALOS PALSAR RTC DEM from 2015 is used in this study. The dataset was developed by the Japan Aerospace Exploration Agency (JAXA) and other collaborators. We downloaded the data on 6 April 2024 from https://asf.alaska.edu/datasets/daac/alos-palsar-radiometric-terrain-correction/ (JAXA et al., 2024).
RBK developed the main idea and wrote the manuscript. SM collected and analyzed the data.
At least one of the (co-)authors is a guest member of the editorial board of Proceedings of IAHS for the special issue “Mountain Hydrology and Cryosphere”. The peer-review process was guided by an independent editor, and the authors also have no other competing interests to declare.
Publisher's note: Copernicus Publications remains neutral with regard to jurisdictional claims made in the text, published maps, institutional affiliations, or any other geographical representation in this paper. While Copernicus Publications makes every effort to include appropriate place names, the final responsibility lies with the authors.
This article is part of the special issue “Mountain Hydrology and Cryosphere”. It is a result of the International Conference on Mountain Hydrology and Cryosphere, Kathmandu and Dhulikhel, Nepal, 9–10 November 2023.
Chen, F., Zhang, M., Tian, B., and Li, Z.: Extraction of glacial Lake outlines in Tibet plateau using Landsat 8 imagery and google earth engine, IEEE J. Sel. Top. Appl., 10, 4002–4009, 2017.
Chikita, K., Jha, J., and Yamada, T.: Hydrodynamics of a supraglacial lake and its effect on the basin expansion: Tsho Rolpa, Rolwaling Nepal Himalaya, Arct. Antarct. Alp. Res., 3, 58–70, 1999.
Froehlich, D. C.: Peak outflow from breached embankment dam, J. Water Res. Pl., 121, 90–97, 1995.
ICIMOD: Glacial lakes and glacial lake outburst floods in Nepal, Kathmandu, ICIMOD, 99 pp., 2011.
Japan Aerospace Exploration Agency (JAXA) and other collaborators: ALOS PALSAR RTC DEM, ASF [data set], https://asf.alaska.edu/datasets/daac/alos-palsar-radiometric-terrain-correction/, last access: 6 April 2024.
Maskey, S., Kayastha, R. B., and Kayastha, R.: Glacial Lakes outburst floods (GLOFs) modelling of Thulagi and lower Barun Glacial Lakes of Nepalese Himalaya, Progress in Disaster Science, 7, 100106, https://doi.org/10.1016/j.pdisas.2020.100106, 2020.
Westoby, M. J., Glasser, N. F., Brasington, J., Hambrey, M. J., Quincey, D. J., and Reynolds, J. M.: Modelling outburst floods from moraine-dammed glacial lakes, Earth Sci. Rev., 134, 137–159, https://doi.org/10.1016/j.earscirev.2014.03.009, 2014.
Zhang, G., Yao, T., Xie, H., Wang, W., and Yang, W.: An inventory of glacial lakes in the Third Pole region and their changes in response to global warming, Global Planet. Change, 131, 148–157, 2015.