the Creative Commons Attribution 4.0 License.
the Creative Commons Attribution 4.0 License.
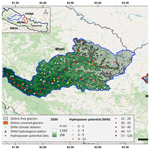
Hydropower potential of the Marsyangdi River and Bheri River basins of Nepal and their sensitivity to climate variables
Rakesh Kayastha
Rijan Bhakta Kayastha
Kundan Lal Shrestha
Smriti Gurung
Understanding the hydrology of the Himalayan region and its response to current and future climate scenarios is crucial for identifying the region's future water availability for infrastructural development. For this, the hydropower potential and the impact of future change on the hydrology of the Bheri River basin (BRb) and the Marsyangdi River basin (MRb) were analysed. The Glacio-hydrological Degree-Day Model version 2.0 (GDM V2.0), developed in the PCRaster dynamic modelling framework, was used to simulate the river runoff. Geospatial tools and different criteria were used to assess topographic features, identify suitable places for run-of-river (ROR) hydropower development, and estimate power potential. Eight scenarios with various combinations of temperature and precipitation changes were used to assess the hydropower potential. Increases in temperature by 0.5 and 1 °C (assumed for the near-term and mid-term future) and changes in precipitation of ±10 % and ±20 %, respectively, are used to conduct a sensitivity analysis for the hydropower potential. A total of 116 and 83 suitable sites were identified, and 4242 and 2823 MW power potentials were estimated in the BRb and the MRb, respectively. All the sensitivity scenarios show an increase in hydropower production, except for one with a drier scenario and less precipitation. The integration of a geographic information system (GIS) and a hydrological model helps us to understand the hydrological response to climate variables and its impact on hydropower in the Himalayan region.
- Article
(2212 KB) - Full-text XML
- BibTeX
- EndNote
Understanding the hydrological characteristics of glacierized catchments is critical for assessing the hydrological dynamics of basins. For communities downstream, these areas provide the majority of the water resources needed for hydropower, irrigation, water supply, and livelihood. Local or global climate variability can have a direct impact on the most efficient use of water resources, particularly hydropower. The variations in climate variables affect the patterns of river runoff, which in turn affects the amount of water available for the production of hydroelectric energy (Immerzeel et al., 2013; Lutz et al., 2014). However, climate change may alter hydrological variability, affecting the water resource systems that depend on the flow regime. Because of the complex connection between variations in the climate, mountainous topography, and hydrological processes and because of the insufficient data, the potential effects of changes in the hydroclimate on hydropower generation are not generally understood, particularly in a Nepalese context. Previously, hydropower infrastructure, design, and operation were estimated using historical streamflow records as a source of information (Shrestha et al., 2014). Shrestha (1966) estimated a total hydropower potential of 83 500 MW in Nepal, with an economically feasible capacity of 45 610 MW from 114 sites. Additionally, a recent study suggested that Nepal has a run-of-river (ROR) hydropower potential of 53 836 MW with a 40 % dependable flow (Jha, 2010; Pradhan, 2009; Shrestha, 1966; WECS, 2011).
Numerous modelling techniques have been developed to enhance comprehension of hydrological dynamics with different levels of complexity. The hydrological model integrated with the geographic information system (GIS) offers a suitable application for hydropower potential evaluation. The hydropower capacity can be estimated more effectively with this integration.
The objective of this study is to assess suitable sites for hydropower development and its power potential in the Bheri River and Marsyangdi River basins using an integrated modelling approach.
This study focuses on the two glacierized sub-basins of the Karnali River and Gandaki River systems, the Bheri River basin (BRb) and the Marsyangdi River basin (MRb), located in western Nepal (Fig. 1). The BRb elevation ranges from 227 to 7598 m above sea level (a.s.l.) with a total area of 13 688 km2, and it is one of the major tributaries of the Karnali River, the third largest river system in Nepal. About 3.93 % (538.71 km2) of the total area of the BRb is glacierized; within that, debris-covered glaciers cover 28.43 km2 (0.02 %). While the MRb is the major tributary of the Gandaki River system, elevation ranges from 258 to 7593 m a.s.l., with a total area of 4786 km2, where 16.75 % (802 km2) of the basin is covered by debris-free glaciers and 0.59 % (28 km2) is covered by debris-covered glaciers. The higher regions of the BRb and MRb lie on the leeward side of mountain ranges (the Dhaulagiri and Annapurna massifs) in the trans-Himalayan region.
The steep slopes and high potential water energy due to the mountainous landscapes in both basins offer opportunities for developing hydropower projects. As of 2023, the Department of Electricity Development (DOED), a government agency in Nepal, has granted licenses for 56 hydropower projects in the MRb. These projects have a combined capacity to generate 2007 MW of electricity. Of these, four hydropower projects in the MRb have a capacity of more than 100 MW of electricity production. In the BRb, 33 hydropower projects have a combined electricity generation capacity of 3934 MW. Notably, 10 of these projects have a capacity exceeding 100 MW. The basin's main rivers, the Marsyangdi and Bheri, have significant hydropower projects because of their consistently high runoff throughout the year.
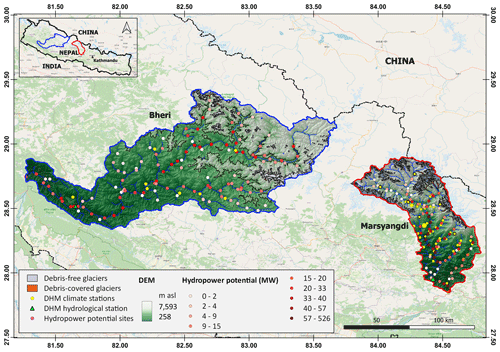
Figure 1Locations of the Marsyangdi River and Bheri River basins in Nepal (Nepal is in the inset). The black line indicates the Nepalese political boundary, the points indicate the hydropower project's potential sites, and the colour ramp indicates its power potential. The green triangle and yellow points represent the hydrological and climatological stations of the Department of Hydrology and Meteorology (DHM) in the MRb and BRb. Background: © OpenStreetMap contributors 2024. Distributed under the Open Data Commons Open Database License (ODbL) v1.0.
3.1 Meteorological and hydrological data
Input data such as daily air temperature, precipitation, and discharge data were obtained from the Department of Hydrology and Meteorology (DHM), Government of Nepal, for the hydrological analysis. The meteorological data with maximum and minimum air temperatures as well as the amount of precipitation were recorded at eight and six stations, respectively, at varying elevations within the basin boundary (Table 1). Daily discharge data from the hydrological stations at Samaijighat at an elevation of 500 m a.s.l. and Bimalnagar at an elevation of 354 m a.s.l. were used for the BRb and the MRb, respectively. The available observed time series meteorological data were used as a forcing dataset for calibrating and validating the hydrological model simulations.
3.2 Spatial data
For the hydrological modelling, Environmental Systems Research Institute (ESRI) Land Use Land Cover (LULC) derived from 10 m resolution Sentinel-2 imagery is obtained from ESRI (https://livingatlas.arcgis.com/landcoverexplorer, last access: November 2022) (Karra et al., 2021) for the land cover information. Ten different class definitions from the ESRI LULC dataset are modified and reclassified with similar topographic features and surface runoff behaviour to create eight land classes for a similar range of rainfall-runoff coefficients. Similarly to this, the Shuttle Radar Topography Mission (SRTM) 1 arcsec global digital elevation model (DEM) with 30 m spatial resolution is obtained from the USGS (https://earthexplorer.usgs.gov, last access: January 2023) and serves as the grid and elevation data for the hydrological model and hydropower potential. Randolph Glacier Inventory Version 6.0 (RGI v6.0) (Arendt et al., 2017) glacier outlines are used for the glacier information and for reclassifying land classes.
3.3 Climate sensitivity
This study considered various scenarios to simulate the potential effects of climate variation on the basins' hydrology. These scenarios were based on changing the temperature and precipitation inputs to the hydrological model following various assumptions about greenhouse gas emissions and their effects. In the Ganga basin, Mishra et al. (2020) projected that temperatures will change by approximately 0.55 ± 0.27 °C in the near future (NF) and by 1.37 ± 0.42 °C in the mid-future (MF), while precipitation is expected to change by about 6.95 ± 7.78 % in the NF and by 15.05 ± 11.3 % in the MF under the SSP245 scenarios.
To represent the NF and MF scenarios, the temperature is increased by 0.5 and 1 °C, respectively. For drier and wetter conditions, precipitation input values are changed by adding and subtracting 10 % and 20 %, respectively, from the baseline values. These temperature and precipitation changes produced eight different climate scenarios (Table 2), which are used as forcing data for the hydrological model and to simulate future hydropower potential.
4.1 Glacio-hydrological model
The Glacio-hydrological Degree-Day Model version 2.0 (GDM V.2) is applied in this study to estimate the discharge of the rivers. It is a distributed glacio-hydrological model developed in the PCRaster modelling framework that can estimate the contributions from snowmelt, ice melt, rain, and baseflow to the river discharge from the glacierized basins (Kayastha et al., 2020; Kayastha and Kayastha, 2019; Khadka et al., 2020; Yang et al., 2022). GDM incorporates a variety of hydrological processes, including groundwater hydrological processes, cryospheric processes, rainfall runoff, and evapotranspiration. The melt module in GDM uses the degree-day approach, a simplified version of the intricate procedure (Braithwaite and Olesen, 1989) for estimating glacier ice melt and snowmelt with the least amount of data needed (Kayastha et al., 2005; Pradhananga et al., 2014). This approach calculated the melts from debris-free, debris-covered and snow above the glaciers as well as snow.
In this study, the 30 m DEM and 10 m LULC were resampled to a 180 m grid, and then GDM was forced for hydrological simulation in both basins. The meteorological data are pre-processed to force the model for the simulation. The daily precipitation is distributed on the resampled grid using the inversed distance weighting (IDW) approach to interpolate precipitation from the meteorological stations to the grid points. The elevation-dependent temperature distribution in the basin is derived by calculating the monthly temperature lapse rate between the reference stations and the highest-elevation station (∗ in Table 1). An environmental lapse rate (−6 °C km−1) is used to distribute temperature above the higher elevation to the rest of the grids.
The threshold temperature determines whether the precipitation is in the form of snow or rain in the grids. The melts from the debris-free and debris-covered glaciers and snow are calculated with the degree-day factor from each grid. Different sets of degree-day factors are applied for each month, covering the range of estimated degree-day factors determined by various glaciers in the Nepalese Himalayas. For the snowmelt and ice-melt processes, two distinct sets of degree-day factors are used; lower factors are applied at elevations below 5000 m, and high factors are applied at elevations above 5000 m. The model accounts for seasonal variations in melting rates, with higher rates in non-monsoon months (reflected by higher degree-day factors) and lower rates during the monsoon.
The daily time series of observed and simulated discharges are compared to determine the model's performance efficiency using the Nash–Sutcliffe efficiency (NSE) and volume difference (VD) indices (Kayastha et al., 2020). The NSE index plays a crucial role in assessing model accuracy in this study. A value exceeding 0.7, coupled with a volume difference within ±10 %, indicates high accuracy and certainty.
4.2 Identification of hydropower sites and potential estimation
The geospatial tools in Quantum Geographic Information System (QGIS) are used to identify potential sites for hydropower projects. The pre-processing steps for the hydrological analysis, such as filling voids, flow directions, and accumulation, were performed for the stream networks and watersheds. The third order of the stream hierarchy with the Strahler methods was evaluated as a potential location for hydropower development, ensuring a consistent water flow. A minimum distance of 7 km between the two consecutive hydropower plants is considered to provide adequate space for civil works and environmental considerations, such as reservoirs, powerhouses, and environmental flows. For the steepness, high gradient, and altitude, an elevation below 4000 m is considered feasible for hydropower project development. The gross head of the stream segments is extracted using the DEM at the initial and final points. In this study, 70 % of the gross head is considered the net or effective head, which will be used for potential estimation. The head greater than 20 m is only considered in this study. The run-of-river hydropower potential is calculated with the following relationship (Eq. 1):
where ρ is the density of water (1000 kg m−3), g is the gravitational acceleration (9.81 m s−2), Q is the discharge (m3 s−1), and h is the net or effective head (m).
The daily runoff at potential sites from the hydrological model is further examined to determine the dependable flow. For a potential hydropower site, the flow exceedance or design discharge is considered at the 40th percentile of dependable flow (Q40), which is calculated using the flow duration curve (FDC).
5.1 Calibration and validation
The model's performance is first evaluated in two river basins by comparing the simulated discharge to the observed discharge in the specific period: from 2004 to 2017 in the BRb and from 2004 to 2010 in the MRb, using observed discharge data from the hydrological station in the basin shown in Fig. 2a and b, respectively. In the BRb, the model's performance is evaluated using 13-year runoff data from the hydrological station at Samaijighat. The model is validated from 2011 to 2017 and calibrated for 6 years from 2004 to 2010. Similarly, the MRb made use of 7 years of runoff data from the Bimalnagar hydrological station. Three years (2008–2010) of observed runoff are used for validation and 4 years (2004–2007) for calibration in the MRb. The NSE and VD metrics of the model's performance indices are used to assess the model's performance. In the BRb and the MRb, the NSEs for the calibration period are 0.82 and 0.81, and the VDs are 4.3 and −5.8, respectively. The NSE for the validation period for the BRb is 0.71, with a VD of −5.3. Similarly, these are 0.7 and 10.6 in the MRb for the validation period. The runoff calibration and validation performance in both of the basins are considered good.
5.2 Suitable sites and hydropower potential
The geospatial analysis identified possible locations for hydropower development in the BRb and the MRb. Overall, 116 potential sites in the BRb and 83 sites in the MRb were identified. Potential sites in the BRb have a catchment area ranging from 17.95 to 865.2 km2, while in the MRb this varies from 14.93 to 316.57 km2. Similarly, in the BRb, the net or effective head varies from 21 to 827 m, whereas in the MRb it varies from 23 to 1034 m.
The gross hydropower potential at each appropriate location was determined using the runoff from the hydrological model simulation. The Q40 from the FDC, the net or effective head, was used to determine the hydropower potential at each appropriate location. According to current climate estimates, the BRb has a gross hydropower production capacity of 4142 MW at 40 % flow exceedance compared to 2823 MW for the MRb. Jha (2010) estimated a 4140 MW capacity in the BRb and a 3251 MW capacity in the MRb using the hydropower model. Similarly, Bajracharya (2015) estimated total potentials of 7755 MW in the BRb and 8257 MW in the MRb using the mean annual flow and 30 % of the dependable flow. WECS (2019) reported higher potentials of 4614 MW in the MRb and 7993 MW in the BRb, which were estimated between the sub-basin level and the adjacent river confluence. This study adopts the concept of cascading, or sequential development, of hydropower projects along rivers and uses the same water flow multiple times to produce electricity.
Although there were only 5 out of 83 potential sites in the MRb, out of 116, 15 potential sites were found to be able to produce more than 100 MW in the BRb. Small hydropower, defined as less than 10 MW, is categorized as 35 in the MRb and as 55 in the BRb. A sensitivity analysis of climate variables revealed that the potential for hydropower increases in all the settings, except for the extremely dry scenario (S8) (Fig. 3).
An integrated approach is used to evaluate hydropower potential and locate potential run-of-river hydropower locations in Nepal's two river basins. As the methodological framework, the GIS and GDM are integrated. The observed daily runoff is used to calibrate and validate the hydrological model at daily time steps, and the NSE and VD indices are used to assess the model's performance. The geospatial criteria and tools in the GIS are used to identify possible locations for hydropower development in the future: 116 and 83 suitable sites are identified in the BRb and MRb, with total hydropower production capacities of 4142 and 2823 MW, respectively. The sensitivity of the hydropower potential is assessed by varying the temperature and precipitation relative to the baseline data. Hydropower production will increase in all the sensitivity scenarios, except for one in which precipitation decreases by 20 %. Hydropower developers, planners of water resources, and legislators can maximize the use of water resources in the Himalayan basin with the help of these findings. Moreover, the study offers insights that can guide future academic investigations into key factors influencing hydropower potential.
The code for the glacio-hydrological model as well as the data supporting the findings of this study are available from the corresponding author on request.
RK: conceptualization, methodology, analysis, writing – original draft. RBK: GDM concept, supervision, review and editing. KLS: GDM development, review and editing. SG: review and editing.
At least one of the (co-)authors is a guest member of the editorial board of Proceedings of IAHS for the special issue “Mountain Hydrology and Cryosphere”. The peer-review process was guided by an independent editor, and the authors also have no other competing interests to declare.
Publisher's note: Copernicus Publications remains neutral with regard to jurisdictional claims made in the text, published maps, institutional affiliations, or any other geographical representation in this paper. While Copernicus Publications makes every effort to include appropriate place names, the final responsibility lies with the authors.
This article is part of the special issue “Mountain Hydrology and Cryosphere”. It is a result of the International Conference on Mountain Hydrology and Cryosphere, Kathmandu and Dhulikhel, Nepal, 9–10 November 2023.
This research has been supported by the University Grant Commission – Nepal (UGC COVID-19 Response, Technical Innovation, Scientific Investigation and Research Grant 2077/78).
Arendt, A., Bliss, A., Bolch, T., et al.: Randolph glacier inventory – A dataset of global glacier outlines: Version 6.0: Technical report, global land ice measurements from space, RGI consortium, https://doi.org/10.7265/4m1f-gd79, 2017.
Bajracharya, I.: Assessment of run-of-river hydropower potential and power supply planning in Nepal using hydro resources, Inst. für Energietechnik und Thermodyn, 7–12, https://doi.org/10.34726/hss.2015.27916, 2015.
Braithwaite, R. J. and Olesen, O. B.: Calculation of Glacier Ablation from Air Temperature, West Greenland, in: Glacier Fluctuations and Climatic Change, Springer, Dordrecht, the Netherlands, 219–233, 1989.
Immerzeel, W. W., Pellicciotti, F., and Bierkens, M. F. P.: Rising river flows throughout the twenty-first century in two Himalayan glacierized watersheds, Nat. Geosci., 6, 742–745, https://doi.org/10.1038/ngeo1896, 2013.
Jha, R.: Total Run-of-River type Hydropower Potential of Nepal, Hydro Nepal J. Water, Energy Environ., 7, 8–13, https://doi.org/10.3126/hn.v7i0.4226, 2010.
Karra, K., Kontgis, C., Statman-Weil, Z., Mazzariello, J. C., Mathis, M., and Brumby, S. P.: Global land use/land cover with Sentinel 2 and deep learning, in: 2021 IEEE international geoscience and remote sensing symposium IGARSS, 4704–4707, https://doi.org/10.1109/IGARSS47720.2021.9553499, 2021.
Kayastha, R. B. and Kayastha, R.: Glacio-hydrological degree-day model (gdm) useful for the himalayan river basins, Himal. Weather Clim. their Impact Environ., Springer, Cham, 379–398, https://doi.org/10.1007/978-3-030-29684-1_19, 2019.
Kayastha, R. B., Ageta, Y., and Fujita, K.: Use of positive degree day methods for calculating snow and ice melting and discharge in glacierized basins in the Langtang Valley, Central Nepal, Climate and Hydrology in Mountain Areas, 7–14, 2005.
Kayastha, R. B., Steiner, N., Kayastha, R., Mishra, S. K., and McDonald, K.: Comparative Study of Hydrology and Icemelt in Three Nepal River Basins Using the Glacio-Hydrological Degree-Day Model (GDM) and Observations From the Advanced Scatterometer (ASCAT), Front. Earth Sci., 7, 354, https://doi.org/10.3389/feart.2019.00354, 2020.
Khadka, M., Kayastha, R. B., and Kayastha, R.: Future projection of cryospheric and hydrologic regimes in Koshi River basin, Central Himalaya, using coupled glacier dynamics and glacio-hydrological models, J. Glaciol., 66, 831–845, https://doi.org/10.1017/jog.2020.51, 2020.
Lutz, A. F., Immerzeel, W. W., Shrestha, A. B., and Bierkens, M. F. P.: Consistent increase in High Asia's runoff due to increasing glacier melt and precipitation, Nat. Clim. Chang., 4, 587–592, https://doi.org/10.1038/nclimate2237, 2014.
Mishra, V., Bhatia, U., and Tiwari, A. D.: Bias-corrected climate projections for South Asia from Coupled Model Intercomparison Project-6, Sci. Data, 7, 1–13, https://doi.org/10.1038/s41597-020-00681-1, 2020.
Pradhan, P. M. S.: Hydropower development, in: The Nepal–India Water Relationship: Challenges, Springer, 125–151, https://doi.org/10.1007/978-1-4020-8403-4_5, 2009.
Pradhananga, N. S., Kayastha, R. B., Bhattarai, B. C., Adhikari, T. R., Pradhan, S. C., Devkota, L. P., Shrestha, A. B., and Mool, P. K.: Estimation of discharge from Langtang River basin, Rasuwa, Nepal, using a glacio-hydrological model, Ann. Glaciol., 55, 223–230, https://doi.org/10.3189/2014AoG66A123, 2014.
Shrestha, H. M.: Cadastre of Potential Hydropower Resources in Nepal, Moscow Power Institute, 1966.
Shrestha, S., Khatiwada, M., Babel, M. S., and Parajuli, K.: Impact of Climate Change on River Flow and Hydropower Production in Kulekhani Hydropower Project of Nepal, Environ. Process., 1, 231–250, https://doi.org/10.1007/s40710-014-0020-z, 2014.
WECS: Water Resources of Nepal in the context of Climate Change, Technical report, Water and Energy Commission Secretariat (WECS), 2011.
WECS: Assessment of Hydropower Potential of Nepal, Technical report, Water and Energy Commission Secretariat (WECS), 2019.
Yang, M., Li, Z., Anjum, M. N., Kayastha, R., Kayastha, R. B., Rai, M., Zhang, X., and Xu, C.: Projection of Streamflow Changes Under CMIP6 Scenarios in the Urumqi River Head Watershed, Tianshan Mountain, China, Front. Earth Sci., 10, 1–14, https://doi.org/10.3389/feart.2022.857854, 2022.