the Creative Commons Attribution 4.0 License.
the Creative Commons Attribution 4.0 License.
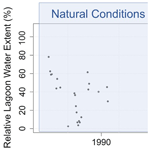
Quantifying irrigation returns into a highly human managed wetland using remote sensing: The Primera de Palos freshwater lagoon (Spain)
Eva Contreras
Rafael Pimentel
Cristina Aguilar
Javier Aparicio
María J. Polo
In this paper, the Global Surface Water Explorer (GSWE) was combined with bathymetric and historical meteorological data to quantify water balance during the period 1984–2020 in the Primera de Palos freshwater lagoon (Southwest Spain). This allowed us, through a water balance approach, to estimate all water inputs and outputs to analyse the hydrological changes in the lagoon. The results showed high fluctuations with seasonal changes marked by the climatic regime during the first two decades of the study period. After this initial period, water extension remained stable above 70 % of the maximum lagoon extent. Thus, the natural hydrological regime of the lagoon was modified by water inputs from irrigation return, which are difficult to quantify. Thanks to a water balance approach, these irrigation returns were quantified as the closure term of the water balance. Three scenarios of irrigation return inputs can be defined, 4500, 1700, and 500 m3 d−1, depending on the cropping season. The use of remote sensing combined with bathymetric and meteorological data can provide the knowledge to support better informed water-management decision-making, although it may have some limitations in dry periods related to image processing in border data.
- Article
(1728 KB) - Full-text XML
- BibTeX
- EndNote
Remote sensing; irrigation returns; freshwater lagoon; human-managed system; UPH 16; Panta Rhei
Wetlands are essential for ecology and environmental regulation (Westerberg et al., 2010; Newton et al., 2018). They also have a key role for the economy and social service functions (Ye and Sun, 2021; Ghanian et al., 2022). However, the natural hydrology of this kind of system is often modified by anthropogenic activities, such as drainage (Sica et al., 2016), mining and drastic modifications of the land uses in the upstream areas (Jia et al., 2013; Li et al., 2021). These changes in hydrological dynamics of these systems, for instance modifications of water fluxes partitioning, are especially relevant in wetlands where their upstream watersheds have a significant proportion of surface altered by these human activities (Merchán et al., 2015). In arid and semi-arid climates, wetlands often rely on inputs from irrigation return flows, which play an important role in water balances of hydrological watersheds (Scott et al., 2011; Merchán et al., 2013). To understand the changes over wetlands ecosystems and their spatiotemporal distribution is critical for the management, restoration, and conservation of these systems.
The development of remote sensing and GIS techniques have emerged as an alternative to traditional methods like field survey (usually laborious, time consuming and expensive) to account for changes. Open-source multispectral satellite remote sensing missions provide worldwide long-term time-series coverage of wetland evolution. There are an increasing number of studies providing evidence about the success of this kind of methods for spatiotemporal monitoring of changes in these habitats (Bortels et al., 2011; Blount et al., 2022; Chen et al., 2022). These techniques can be also used to analyse the effects of anthropogenic activities in the water bodies.
An example of a human-modified freshwater lagoon due to agricultural pressure is the Primera de Palos lagoon, which is located in a highly modified marshy environment in southwestern Spain. In this study we aim to analyse the changes in the lagoon hydrological dynamics during the last thirty years narrowing down the ranges of the different water fluxes involved in the water balance and accounting specifically from irrigation returns. For that, remote sensing information in combination to meteorological data and the bathymetry of the lagoon were used. This work examines research question No 16 of the twenty-three unsolved problems in hydrology (UPH; Blöschl et al., 2019): “How can we use innovative technologies to measure surface and subsurface properties, states, and fluxes, at a range of spatial and temporal scales?”
2.1 Primera de Palos freshwater lagoon
In this work we analysed the evolution of the Primera de Palos freshwater lagoon (Southwest Spain) (Fig. 1), a Ramsar site whose surrounding lands have been intensively modified by petrochemical industry and greenhouse strawberry crops. This water body is part of a complex wetland that has its origin in the closure of old valleys or riverbeds due to the advance of a coastal dune front that acts as a barrier for both, the inland water flowing to the sea and the sea water flowing into the lagoon avoiding saline intrusion (Mantecon et al., 1988). The contributing watershed, which is entirely dedicated to strawberry cultivation from 1995, is made up of a sandy substrate that allows irrigation water to drain into the wetland.
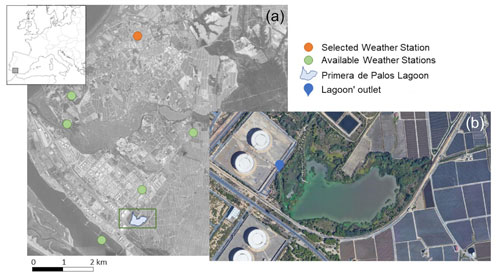
Figure 1(a) Geographic location of the Primera de Palos lagoon in the Southwestern Spain. (b) Zoom to the Primera de Palos lagoon. © Google 2023 Primera de Palos freshwater lagoon. Available at: https://www.google.com/ (last access: 30 March 2023).
2.2 Data
We have used different sources of data in this study. On the one hand, since there are no historical series of flow measurements in the lagoon or in its contributing watershed, the Global Surface Water Explorer (GSWE), a remote sensing based virtual online machine was used to spatially quantify water surface during the historical period 1984–2020 (Pekel et al., 2016). The explorer provides extent and changes over time of the world's water extensions at 30 m spatial resolution. Since our study goes until 2022, Landsat information from the period 2020–2022 was used for deriving the lagoon extension using the Normalized Water Difference Index (NWDI, Gao, 1996). This information was combined with bathymetric data (provided by TYPMA S.L, a consultancy firm which participates in some management tasks in the wetland) and historical meteorological data from the weather station close to the lagoon with the longest dataserie – Palos de la Frontera (provided by the Spanish State Meteorological Agency).
In addition, from May 2021 to March 2022, periodic measurements of streamflow and water level in the lagoon outlet (Fig. 1) were carried out with a weekly/biweekly frequency (Table 1). These observations will also allow us to establish a limit value of the water surface from which there is no outflow in the lagoon.
A water balance approach was carried out to estimate the lagoon dynamics:
where, Δt is the variation of time between consecutives dates with available satellite information, is the variation of volume in the lagoon in each time interval, P accounts for precipitation in the lagoon, Qr is the direct runoff into the lagoon, E is the evapotranspiration from the lagoon, Id represents the subsurface flow, R accounts for the irrigation returns and Qout accounts for water outflow. Each term of the balance equation was computed as followed:
-
is calculated as the difference between the water volumes between two consecutive images. For each date, the volume of the lagoon was derived using the water surface from the remote sensing information and the relationship between water surface – water volume – lagoon wet area – elevation, established using the bathymetric information.
-
P was estimated as the product of the accumulated precipitation in each interval and the average area of the water surface of the lagoon between images.
-
Qr, was estimated as the product of the accumulated precipitation, which is assumed constant over the drainage area into the lagoon, this contributing area (3.73 km2) and a variable runoff coefficient (c) over time based on the main land use on this area. We assumed a c=0.31 for no crops under plastic and c=0.50 for crops under plastic.
-
E, was estimated as the product of the potential evaporation accumulated between images and the average area of the water surface of the lagoon between these days. The potential evaporation on a daily scale was calculated from minimum, mean and maximum temperature data using the Hargreaves equation (Hargreaves and Samani, 1985). IdR and Qout cannot be directly estimated. Therefore, they are grouped in one term and considered the closure term of the water balance.
To account for each of these individual terms we analysed specific situations in which some of the terms can be considered null.
-
Situation A – Natural conditions: It assumes that there was no relevant irrigation contribution to the lagoon (R=0) and a negligible value of the outflow (Qout=0), since the water levels in the lagoon were lower than the height of the outlet. The closure term becomes Id in this case.
-
Situation B – Modified conditions: Under this situation the closure term will be the sum of R, Qout and Id. However, analysing each of the water fluxes dynamics we can understand seasonal dynamics of this term. We assume that this period is representative of the current lagoon state in terms of water balance.
-
Situation C – Year 2021–2022: We have measurements of Qout during the last year. Then the closure term can be reduced to R+Id. If we have obtained the Id estimates for the situation A and that estimates are kept in time. We can provide a value for the irrigation returns into the lagoon.
The analysis of the GSWE' water extension during the analysed period 1984–2020 (Fig. 2), showed high fluctuations during the first two decades of the study period. These fluctuations are clearly linked to the climatic regime, that is, the lagoon was emptier in dry years than in wet years. However, from 1995 linked to a boom in the use of the greenhouse for strawberry crops, the water extension in the lagoon did not show these high fluctuations. Since then, the water extension of the lagoon has always been above 70 % of the maximum lagoon extent (121 149 km2). These results allowed us to verify the hypothesis of a modification in the lagoon dynamics due to the strawberry crop irrigation. Therefore, the period that represents Situation A – natural conditions goes from 1984 until 1995 and the period representing Situation B – modified conditions goes from 1996 until 2022.
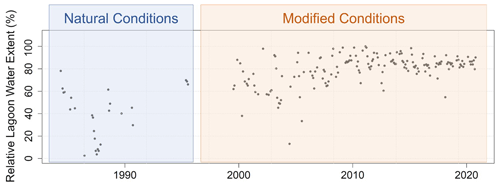
Figure 2Evolution of the percentage of relative lagoon water surface with respect to the maximum extension value for the period 1984–2020.
4.1 Situation A – Natural conditions
For this first period 1984–1995, the number of satellite images was low, just 20 dates with available information (Table 2). This fact makes the Δt use in the balance too long, diluting the outcomes of the analysis. In addition, the images also coincide with very dry months, usually in summer, during the analysed period. Consequently, the water extensions in the lagoon were very reduced. This makes errors in the retrieving process to become higher. That is, most of the pixels identified as water were susceptible to be erroneous due to border effect. Previous studies (Blount et al., 2022) already pointed out that the main source of error in the satellite results is the dataset spatial resolution limits, thus, in this case, the error associated with the volume calculation could be relevant. For this reason, we decided to rule out the water balance estimates for this period. However, the images analysed showed that the water levels in the lagoon decrease during all year except for winter months (Table 2). Therefore, we can assume that there was no inflow into the lagoon and thus under natural conditions we can assume that Id=0 for this period. In addition, for these months, the water extent in the lagoon was always below 70 % of its maximum lagoon extent (Fig. 2).
4.2 Situation B – Modified conditions
Figure 3 shows the monthly evolution of the different terms of the water balance in the lagoon for the period 1996–2020. In this case the closure term refers to the sum of subsurface flow, irrigation return and outflow (). A negative value of this term indicates that outflows from the lagoon (Qout) are greater than inflows (due to Id and R) into the lagoon. These conditions were found from November to May (Fig. 4). Usually in this period, water extension in the lagoon presents higher values, always above 70 % of the maximum lagoon extent (Fig. 2). In addition, these months also coincide with the strawberry crops' irrigation period. On the other hand, results showed positive values of the closure term from June to October (Fig. 4). That implies that during these months Qout is almost neglectable.
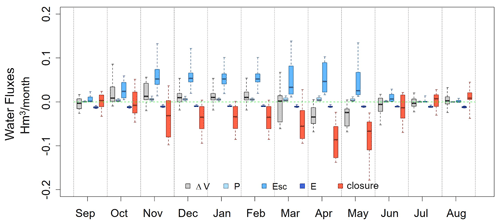
Figure 3Monthly evolution of the different terms of the water balance in the Primera de Palos lagoon for the period 1996–2020.
4.3 Situation C – Year 2021–2022
The Qout measured in the outlet of the lagoon (Table 2) helped us to verify the outcomes obtained about seasonality in the lagoon. On the one hand, outflow dynamic follow the pattern previously defined. Qout decreases from May to June, it is null from June to November and increases from November on. This last increase of Qout follows two different rates (about 10 from November to March and about 500 from March to May). On the other hand, we have been able to verify the threshold that defines when the lagoon is draining out (70 % of the maximum lagoon extent).
Moreover, having Qout values along this period allows us to reduce the closure term to the sum of Id+R. Figure 4 shows the evolution of this term for the days when satellite images were available during the period with outflow measurements. According to our hypothesis of Id=0, except for winter, the closure term is equivalent to R.
Under these conditions, we were able to account for the irrigation return inputs into the lagoon establishing three possible scenarios: (i) high irrigation return inflow, about 4500 m3 d−1 (in April); (ii) medium irrigation return inflow, about 1700 m3 d−1 (July, October, and December); and low irrigation return inflow, about 500 m3 d−1 (June and November).
For the higher contribution rate, assuming an irrigation period about 100 d yr−1 and a water volume allocation for strawberries irrigation of 7000 m3 ha−1, the irrigation volume allocated for strawberries in the watershed would be about 700 mm yr−1, which means that a 15 % of the total irrigation volume is reaching the lagoon.
This study quantifies the hydrological dynamics of a highly human managed wetland in southwestern Spain. The case study of the Primera de Palos freshwater lagoon can be seen as a pilot area for testing a methodology to account for unmeasurable water fluxes in a lagoon water balance approach. The main findings of this work can be summarised:
-
The remote sensing product used, GWSE, combined with local information, can be used to successfully evaluate hydrological behaviour in water bodies when historical hydrological data series are not available.
-
The spatiotemporal resolution of GWSE has proven to be enough to capture seasonal dynamics of water fluxes in the analysed lagoon. Only, some limitations have been highlighted connected to low levels in the lagoon. This small water extent can be linked to a wrong water pixel identification. In this situation the use of higher resolution remote sensing data, as Sentinel-2 will be the solution.
-
The methodology applied, identifying key situations in which some terms in the water balance equation can be neglected, allowed us to define the behaviour of the water surface for the period 1984–2020.
-
Three scenarios of irrigation return were defined according to the season. The quantification of the magnitude of all water fluxes helped to not only reduce the uncertainty regarding the unmeasured water fluxes but also to establish the basis for a water balance model to better inform water-management decision-making.
The software is available from the corresponding author, Eva Contreras, upon reasonable request.
In relation to the data, two different Earth Observation (EO) products were used for this research: (1) Global Surface Water Explorer (Pekel et al., 2016) available at https://global-surface-water.appspot.com/download (last access: 1 June 2022) and (2) Landsat 8 scenes, available at https://earthexplorer.usgs.gov/ (USGS, 2022). Meteorological dataset, Palos de la Frontera station is available at https://www.aemet.es/es/datos_abiertos (AEMET, 2022). In situ measurements of outflow and water level are available in Table 1. Bathymetric information is not publicly available due to privacy restriction.
EC: Conceptualization, Data curation analysis, Formal analysis, Investigation, Methodology, Software, Visualization, Writing – original draft preparation.
RP: Conceptualization, Data curation, Formal analysis, Investigation, Methodology, Software, Visualization, Writing – review & editing.
CA: Methodology, Writing – review & editing.
JA: Data curation, Writing – review & editing.
MJP: Conceptualization, Funding acquisition, Supervision, Methodology, Writing – review & editing.
The contact author has declared that none of the authors has any competing interests.
Publisher’s note: Copernicus Publications remains neutral with regard to jurisdictional claims in published maps and institutional affiliations.
This article is part of the special issue “IAHS2022 – Hydrological sciences in the Anthropocene: Past and future of open, inclusive, innovative, and society-interfacing approaches”. It is a result of the XIth Scientific Assembly of the International Association of Hydrological Sciences (IAHS 2022), Montpellier, France, 29 May–3 June 2022.
Rafael Pimentel acknowledges fundings by the Juan de la Cierva In corporación Programme of the Spanish Ministry of Science and Innovation (IJC2018–038093-I). Javier Aparicio acknowledges fundings by the Programme Ayudas para contratos predoctorales para la formación de doctores of the Spanish Ministry of Science and Innovation (PRE2019–090493). Eva Contreras, Rafael Pimentel, Javier Aparicio and María J. Polo are members of DAUCO, Unit of Excellence ref. CEX2019–000968-M, with financial support from the Spanish Ministry of Science and Innovation, the Spanish State Research Agency, through the Severo Ochoa and María de Maeztu Program for Centers and Units of Excellence in R&D.
This research has been supported by the environmental consulting company Tratamiento Proyectos Medioambientales, S.L (Analysis of the hydrological viability of the drainage actions in the Primera de Palos freshwater lagoon).
This paper was edited by Christophe Cudennec and reviewed by two anonymous referees.
AEMET: AEMET Open Data, AEMET [data set], https://www.aemet.es/es/datos_abiertos, last access: 30 June 2022.
Blöschl, G. et al.: Twenty-three unsolved problems in hydrology (UPH) – a community perspective, Hydrol. Sci. J., 64, 1141–1158, https://doi.org/10.1080/02626667.2019.1620507, 2019.
Blount, T. R., Carrasco, A. R, Cristina, S., and Silvestri, S.: Exploring open-source multispectral satellite remote sensing as a tool to map long-term evolution of salt marsh shorelines, Estuar. Coast Shelf S., 266, 107664, https://doi.org/10.1016/j.ecss.2021.107664, 2022.
Bortels, L., Chan, J. C.-W., Merken, R., and Koedam, N.: Long-term monitoring of wetlands along the Western-Greek Bird Migration Route using Landsat and ASTER satellite images: Amvrakikos Gulf (Greece), J. Nat. Conserv., 19, 215–223, https://doi.org/10.1016/j.jnc.2011.01.004, 2011.
Chen, K., Cong, P., Qu, L., Liang, S., and Sun, Z.: Annual variation of the landscape pattern in the Liao River Delta wetland from 1976 to 2020, Ocean Coast. Manage., 224, 106175, https://doi.org/10.1016/j.ocecoaman.2022.106175, 2022.
Hargreaves, G. H. and Samani, Z. A.: Reference Crop Evapotranspiration from Temperature, Appl. Eng. Agric., 1, 96–99, https://doi.org/10.13031/2013.26773, 1985.
Gao, B.-C.: NDWI – A normalized difference water index for remote sensing of vegetation liquid water from space, Proc. SPIE, 58, 257–266, https://doi.org/10.1016/S0034-4257(96)00067-3, 1996.
Ghanian, M., Ghoochani, O. M., Noroozi, H., and Cotton, M.: Valuing wetland conservation: A contingent valuation analysis among Iranian beneficiaries, J. Nat. Conserv., 66, 126140, https://doi.org/10.1016/j.jnc.2022.126140, 2022.
Jia, Z., Wu, Z., Luo, W., Xi, W., Tang, S., Liu, W. L., and Fang S.: The impact of improving irrigation efficiency on wetland distribution in an agricultural landscape in the upper reaches of the Yellow River in China, Agr. Water Manage., 121, 54–61, https://doi.org/10.1016/j.agwat.2013.01.003, 2013.
Li, S., Wu, M., Jia, Z., Luo, W., Fei, L., and Li, J.: Study on drainage strategy of ditch wetland in semi-arid area under the influence of inflow from the upstream irrigation area, Agr. Water Manage., 248, 106792, https://doi.org/10.1016/j.agwat.2021.106792, 2021.
Mantecon-Gómez, R., Martín-Machuca, M., and Mediavilla-Laso, C.: Problemas de sobreexplotación e intrusión marina en los acuíferos costeros del litoral atlántico andaluz, 504–520, in: Tecnología de la intrusión de los acuíferos costeros, edited by: Fernández-Rubio, R., López Geta, J. A., and Ramos González, G., TIAC, 88, 654 pp., 1988.
Merchán, D., Causapé, J., and Abrahão, R.: Impact of irrigation implementation on hydrology and water quality in a small agricultural basin in Spain, Hydrolog. Sci. J., 58, 1400–1413, https://doi.org/10.1080/02626667.2013.829576, 2013.
Merchán, D., Causapé, J., Abrahão, R., and García-Garizábal, I.: Assessment of a newly implemented irrigated area (Lerma Basin, Spain) over a 10-year period. I: Water balances and irrigation performance, Agr. Water Manage., 158, 277–287, https://doi.org/10.1016/j.agwat.2015.04.016, 2015.
Newton, A., Brito, A. C., Icely, J. D., Derolez, V., Clara, I. Angus, S., Schernewski, G., Inácio, M., Lillebø, A. I., Sousa, A. I., Béjaoui, B., Solidoro, C., Tosic, M., Cañedo-Argüelles, M., Yamamuro, M., Reizopoulou, M., Tseng, S., Canu, H.-C., Roselli, D., Maanan, L., Cristina, S., Ruiz-Fernández, A. C., de Lima, R. F. d., Kjerfve, B., Rubio-Cisneros, N., Pérez-Ruzafa, A., Marcos, C., Pastres, R., Pranovi, F., Snoussi, M., Turpie, J., Tuchkovenko, Y., Dyack, B., Brookes, J., Povilanskas, R., and Khokhlov, V.: Assessing, quantifying and valuing the ecosystem services of coastal lagoons, J. Nat. Conserv., 44, 50–65, https://doi.org/10.1016/j.jnc.2018.02.009, 2018.
Pekel, J. F., Cottam, A., Gorelick, N., and Belward, A. S.: High-resolution mapping of global surface water and its long-term changes, Nature, 540, 418–422, https://doi.org/10.1038/nature20584, 2016 (data available at: https://global-surface-water.appspot.com/download, last access: 1 June 2022).
Scott, J., Rosen, M. R., Saito, L., and Decker, D. L.: The influence of irrigation water on the hydrology and lake water budgets of two small arid-climate lakes in Khorezm, Uzbekistan, J. Hydrol., 410, 114-125, https://doi.org/10.1016/j.jhydrol.2011.09.028, 2011.
Sica, Y. V., Quintana, R. D., Radeloff, V. C., and Gavier-Pizarro, G. I.: Wetland loss due to land use change in the Lower Paraná River Delta, Argentina, Sci. Total Environ., 568, 967–978, https://doi.org/10.1016/j.scitotenv.2016.04.200, 2016.
USGS: Landsat 8 scenes, USGS [data set], https://earthexplorer.usgs.gov/, last access: 1 June 2022.
Westerberg, V. H., Lifran, R., and Olsen, S. B.: To restore or not? A valuation of social and ecological functions of the Marais des Baux wetland in Southern France, Ecol. Econ., 69, 2383–2393, https://doi.org/10.1016/j.ecolecon.2010.07.005, 2010.
Ye, C. and Sun, F.: Development of a social value evaluation model for coastal wetlands, Ecol. Inform., 65, 101417, https://doi.org/10.1016/j.ecoinf.2021.101417, 2021.