the Creative Commons Attribution 4.0 License.
the Creative Commons Attribution 4.0 License.
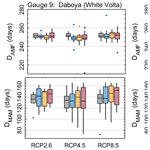
Future shifting of annual extreme flows under climate change in the Volta River basin
Mathieu Vrac
Natalie Ceperley
Sander J. Zwart
Josh Larsen
Simon J. Dadson
Grégoire Mariéthoz
Bettina Schaefli
Global warming is projected to result in changes in streamflow in West Africa with implications for frequent droughts and floods. This study investigates projected shifting in the timing, seasonality and magnitude of mean annual minimum (MAM) and annual maximum flows (AMF) in the Volta River basin (VRB) under climate change, using the method of circular statistics. River flow is simulated with the mesoscale hydrologic model (mHM), forced with bias-corrected climate projection datasets consisting of 43 regional and global climate model combinations under three representative concentration pathways (RCPs). Projected changes indicate that AMF increases between +1 % and +80 % across sub-basins, particularly in the near future (2021–2050), whereas MAM decreases between −19 % and −7 %, mainly from the late century (2071–2100), depending on RCPs. The date of occurrence of AMF is projected to change between −4 and +3 d, while MAM could shift between −4 and +14 d depending on scenarios over the 21st century. Annual high flows denote a strong seasonality with negligible future changes, whereas the seasonality of low flows has a higher variation, with a slight drop in the future.
- Article
(2234 KB) - Full-text XML
- BibTeX
- EndNote
Hydroclimatic extremes; modelling; West Africa; PUB; UPH 1; UPH 9; SDG 6.4; SDG 13.1
Human-induced climate warming has significantly altered the magnitude of mean and extreme river flows globally (Gudmundsson et al., 2021). River flow is a key indicator of water availability for people and the environment. Fluctuations in river water availability can undermine water supply and profoundly affect water and food security. Therefore, knowing trends in river flows is crucial for preserving livelihoods and safeguarding ecosystems. In West Africa, global warming has induced a significant increase in extreme rainfall, thereby affecting river flows (Chagnaud et al., 2022; Rameshwaran et al., 2021). Streamflow extremes in West Africa are non-stationary and associated with devastating floods and droughts that are becoming persistent and widespread (Elagib et al., 2021; Wilcox et al., 2018). As flood and drought are two extremes of the same hydrological cycle, it is important to integrate them in disaster reduction strategies and measures to better cope with current and future risks (Ward et al., 2020; Brunner et al., 2021; Kreibich et al., 2022). Understanding future dynamics in the occurrence of annual extreme river flows is critical to guide adaptation measures (Lane and Kay, 2021). However, studies usually focus on assessing changes in the magnitude of river flows while timing and seasonality are also important for water planning and management.
This study investigates the shifting of annual minimum and maximum flows timing, seasonality and magnitude using a large ensemble of regional and global climate models under multiple climate change scenarios in the Volta River basin (VRB), where continuous updates on the state of water resources is essential to cope with persisting water-related risks. Here, annual minimum and maximum flows are analysed instead of flow percentiles as done by Dembélé et al. (2022).
The Volta River Basin (VRB) covers approximately 415 600 km2 shared among six countries (Benin, Burkina Faso, Côte d'Ivoire, Ghana, Mali, and Togo) in West Africa (Fig. 1). The land cover is dominated by savannah, with a maximum altitude of 940 m a.s.l. Rainfall is characterized by interannual and multidecadal variabilities, and ranges between 570 and 1200 mm yr−1 from north to south. Actual evaporation exceeds 80 % of the basin annual rainfall. The Black Volta (152 800 km2), White Volta (113 400 km2), Oti (74 500 km2) and Lower Volta (74 900 km2) constitute the four sub-basins of the drainage system. The Volta River flows over 1850 km and fills in the Lake Volta formed by the Akosombo dam before draining into the Atlantic Ocean (Dembélé, 2020).
3.1 Climate projection data
Five Regional Climate Models (RCMs) from the Coordinated Regional-climate Downscaling Experiment (CORDEX) for Africa at a spatial resolution of 0.44° (∼ 50 km) are considered with twelve General Circulation Models (GCMs) from the fifth Coupled Model Intercomparison Project (CMIP5; Taylor et al., 2012) under three Representative Concentration Pathways (RCPs; Van Vuuren et al., 2011), resulting in 43 RCM-GCM combinations. The daily data include rainfall and air temperature (average, maximum and minimum). The historical runs consider 21 RCM-GCM combinations, whereas the future projections involve 18 RCM-GCM combinations for RCP8.5, 16 for RCP4.5 and 9 for RCP2.6 (Table 1). The climate projection datasets are evaluated with the best-performing satellite and reanalysis rainfall and temperature products in the VRB (Dembélé et al., 2020c), after a multivariate bias correction with the Rank Resampling for Distributions and Dependences (R2D2) method (Vrac and Thao, 2020).
3.2 Hydrological modelling
The fully distributed mesoscale Hydrologic Model (mHM) (Kumar et al., 2013; Samaniego et al., 2010) is used to simulate daily streamflow, using the bias corrected climate projection datasets as input. The model configuration and performance are given by Dembélé et al. (2020b). The periods 2021–2050, 2051–2080 and 2071–2100, representing the near-term future, the long-term future and the late-century, are considered to assess the impact of climate change on streamflow, relative to the historical period or baseline (1991–2020). The detailed methodology and the bias-correction results are given by Dembélé et al. (2022).
3.3 Timing of high and low flows
The mean annual minimum flow (MAM) of seven consecutive days is considered as low flow, while high flow is the annual maximum flow (AMF) corresponding to the highest peak flow in a calendar year. High and low flows are estimated at Bui-Amont, Daboya and Saboba, which correspond to the outlets of the Black Volta, White Volta and Oti sub-basins (Fig. 1). The method of circular statistics (Mardia, 1972; Fisher, 1993) is used to estimate the timing of AMF and MAM based on the mean date of occurrence (D) and their seasonality based on the concentration of the dates of occurrence (R) for each of the 30-year historical and future periods (e.g., Blöschl et al., 2017; Hanus et al., 2021; Villarini, 2016). Julian dates are converted into angles that represent locations on the circumference of a circle to avoid concerns with calculating the mean when the day of occurrence comes around the start or end of a calendar year (Young et al., 2000). The angular value of the date of occurrence is obtained as follows:
Where Di is the Julian date of occurrence of AMF or MAM varying between 1 and 365 (366 for leap years), mi represents the number of days in a calendar year i, and θi is the angular date of occurrence in radians.
The mean date of occurrence is calculated as:
with
where is the average number of days in a year, n represents the total number of years and and correspond to the cosine and sine components of the mean date, respectively.
The mean resultant R gives the concentration of the dates of occurrence around the average date as follows:
A small value of R close to 0 suggests a high interannual variability of the date of occurrence, whereas R values near 1 denote a high seasonality in the date of occurrence.
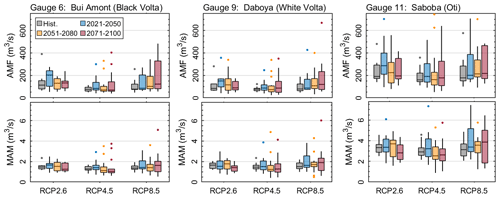
Figure 2Mean annual minimum flows (MAM) and annual maximum flows (AMF) in the major sub-basins of the VRB (Black Volta, White Volta, Oti) over the historical (1991–2020) and future periods.
4.1 Changes in the magnitude of annual extreme flows
Considering all RCPs over the historical period, the average multi-model ensemble medians of MAM and AMF vary across sub-basins in the VRB as follows (Fig. 2): Black Volta (AMF = 88.6 ± 17 m3 s−1, MAM = 1.4 ± 0.1 m3 s−1), White Volta (AMF = 79.3 ± 3 m3 s−1, MAM = 1.5 ± 0.1 m3 s−1) and Oti (AMF = 176.1 ± 12 m3 s−1, MAM = 3.1 ± 0.2 m3 s−1). However, large differences are found in AMF across RCPs, with the highest values occurring under RCP2.6 and the lowest values under RCP4.5.
Future changes in annual high flows indicate an increase in median AMF in all the sub-basins under RCP2.6 and RCP8.5 over the 21st century, and also under RCP4.5 except over 2051–2080 in the White Volta and over 2051–2100 in the Black Volta. The increase in median AMF varies between +1 % in the Oti under RCP4.5 over 2051–2080 and +80 % in the White Volta under RCP2.6 over 2021–2050 with 56 % agreement on the direction of change among the RCM-GCMs. It is noteworthy, that AMF is projected to increase under all scenarios in the near future 2021–2050 in all sub-basins, with +11 % increase on average under RCP8.5, +15 % under RCP4.5 and +70 % under RCP2.6, with 76 %, 71 % and 59 % agreement on the direction of change of RCM-GCMs, respectively. An increase in flood occurrence is projected in all sub-basins under RCP2.6 and RCP8.5 over the 21st century, and mainly over 2021–2050 under RCP4.5.
For annual low flows, the projected decrease in median MAM varies between −19 % in the Black Volta under RCP4.5 over 2071–2100 and −7 % in the White Volta under RCP2.6 over 2021–2050. An overall median increase of MAM in all the sub-basins is projected under RCP8.5 by +16 % in the White Volta, +15 % in the Oti and +9 % in the Black Volta. Under RCP4.5, median MAM increases during 2021–2050 and decreases afterwards by −6 % on average in all sub-basins, whereas it increases until 2080 under RCP2.6 before decreasing by −15 % on average in the late 21st century. It is noteworthy that the median MAM increases under RCP2.6 by +8 % in the White Volta during 2051–2080. The agreement on the direction of change between the RCM-GCMs is on average 65 % for RCP8.5, 62 % for RCP4.5 and 59 % for RCP2.6. A higher likelihood for river droughts is projected from 2051 under RCP4.5 and from 2071 under RCP2.6 in all sub-basins.
4.2 Changes in the timing and seasonality of annual extreme flows
The median date of occurrence of AMF(DAMF) varies on average between the Julian calendar days 246 and 252 (first dekad of September) over the historical period in all sub-basins, and it is projected to decrease by −2 d over the 21st century for all RCPs (Fig. 3). However, the highest reductions per sub-basin are −4 d in the Black Volta over 2051–2080 and −3 d in the White Volta and Oti over 2021–2050, all under RCP4.5. However, a rise by +3 d is projected in the White Volta over 2051–2080 under RCP8.5. Contrastingly, the median DMAM varies between 132 and 139 d (second dekad of May) over the historical period and increases on average by +6 d across sub-basins during the 21st century. However, notable rises in DMAM are projected with +14 d under RCP8.5 over 2071–2100 in the White Volta, +11 d under RCP4.5 over 2051–2080 in the Black Volta and +10 d under RCP2.6 over 2071–2100 in the Oti, which might be the consequence of the forward shift of the rainy season (Dembélé et al., 2022). However, it is notable that DMAM is projected to drop by −4 d over 2021–2050 under RCP8.5 in the Oti and the Black Volta.
The concentration of the date of occurrence (R) of DAMF demonstrates a high seasonality in the occurrence of AMF (RAMF=0.97) across sub-basins, and hardly changes over the 21st century (Fig. 4). The maximum change in RAMF is −3 % over 2071–2100 in the White Volta under RCP8.5. The median RMAM is 0.58 on average across sub-basins over the historical period and slightly decrease in the future, denoting a lower seasonality of MAM. A future reduction in RMAM is observed in the Black Volta (−20 % to −2 %) and Oti (−22 % to −1 %) for all future climate scenarios, while changes are contrasted in the White Volta (−15 % to +6 %).
The large spread of AMF and MAM over 2071–2100 (Fig. 2), denoted by the moderate agreement on the direction of change of RCM-GCM combinations, can be explained by the limited capability of the models to project future trends, in addition to uncertainties associated with the hydrological model. Attention is solely given to climate-induced changes in river flow because climate was found to be the key driver in changes to global river flow (Gudmundsson et al., 2021). However, more local and regional changes in river flow might also result from other factors such as land and water management. Consequently, future studies in the VRB should also consider human interactions with the hydrological cycle (e.g. urbanization, agriculture, reservoirs) to better investigate the causes of changes in river flows (Yang et al., 2021; Yonaba et al., 2021). Furthermore, there is a need to improve hydrological modelling at large scale with earth observation data to better predict the spatial and temporal occurrence of extreme events (Dembélé et al., 2020a; Lindersson et al., 2020). Finally, uncertainties in hydrological modelling could be addressed by adopting multi-model approaches (Giuntoli et al., 2015; Vetter et al., 2017).
The analysis of projected shifting in timing, seasonality and magnitude of mean annual minimum flows and annual maximum flows in the Volta River basin under climate change reveals various changes over the 21st century. In general, projected annual high flows increase in all the sub-basins, particularly under RCP2.6 and RCP8.5, thereby leading to higher flood probability. Increase in river droughts are projected from 2051 under RCP4.5 and from 2071 under RCP2.6 in all sub-basins. High flows have a high seasonality with a mean date of occurrence that hardly changes in the future as opposed to low flows that show a future forward shift in time. These findings provide an important basis to inform research and climate change adaptation and mitigation strategies in the Volta River basin.
The source code of the mHM model can be accessed at https://doi.org/10.5281/zenodo.1299584 (Samaniego et al., 2018).
CORDEX data is available from the Earth System Grid Federation database at https://esgf-data.dkrz.de (ESGF, 2020). The database of the hydrological modelling is available at https://doi.org/10.5281/zenodo.3531873 (Dembélé, 2019).
MD designed the experiment, performed the analyses and drafted the paper. All co-authors contributed to the writing, review and editing.
The contact author has declared that none of the authors has any competing interests.
Publisher’s note: Copernicus Publications remains neutral with regard to jurisdictional claims in published maps and institutional affiliations.
This article is part of the special issue “IAHS2022 – Hydrological sciences in the Anthropocene: Variability and change across space, time, extremes, and interfaces”. It is a result of the XIth Scientific Assembly of the International Association of Hydrological Sciences (IAHS 2022), Montpellier, France, 29 May–3 June 2022.
We thank the streamflow data providers: Volta Basin Authority (VBA), Direction Générale des Ressources en Eau (DGRE) of Burkina Faso, Hydrological Services Department (HSD) of Ghana and the Direction Générale de l'Eau et de l'Assainissement (DGEA) of Togo. We are grateful to the developers of the global and regional climate models of CORDEX. We thank the developers of mHM at CHS/UFZ (Germany).
Moctar Dembélé received funding by the Swiss Government Excellence Scholarship (grant no. 2016.0533/Burkina Faso/OP), the Swiss National Science Foundation (SNSF) Doc.Mobility fellowship (SNF, grant no. P1LAP2_178071), the Hydro-JULES visiting scientist fellowship (UKCEH; grant no. NERC NE/S017380/1) and the Sivapalan Young Scientists Travel Award (SYSTA) for participation to the 2022 IAHS Scientific Assembly. Bettina Schaefli was supported by a research grant of the SNSF (SNF, PP00P2_157611). Mathieu Vrac was supported by the COESION project funded by the French National program LEFE (Les Enveloppes Fluides et l'Environnement).
This paper was edited by Christophe Cudennec and reviewed by Jean-Marie Kileshye-Onema and one anonymous referee.
Blöschl, G., Hall, J., Parajka, J., Perdigão, R. A., Merz, B., Arheimer, B., Aronica, G. T., Bilibashi, A., Bonacci, O., and Borga, M.: Changing climate shifts timing of European floods, Science, 357, 588–590, https://doi.org/10.1126/science.aan2506, 2017.
Brunner, M. I., Slater, L., Tallaksen, L. M., and Clark, M.: Challenges in modeling and predicting floods and droughts: A review, WIRES Water, 8, e1520, https://doi.org/10.1002/wat2.1520, 2021.
Chagnaud, G., Panthou, G., Vischel, T., and Lebel, T.: A synthetic view of rainfall intensification in the West African Sahel, Environ. Res. Lett., 17, 044005, https://doi.org/10.1088/1748-9326/ac4a9c, 2022.
Dembélé, M.: Database for the manuscript “Improving the predictive skill of a distributed hydrological model by calibration on spatial patterns with multiple satellite datasets”, Zenodo [data set], https://doi.org/10.5281/zenodo.3531873, 2019.
Dembélé, M.: Spatially explicit hydrological modelling for water accounting under climate change in the Volta River Basin in West Africa, PhD, University of Lausanne, Lausanne, Switzerland, 271 pp., https://doi.org/10.13140/RG.2.2.15664.58885, 2020.
Dembélé, M., Ceperley, N., Zwart, S. J., Salvadore, E., Mariéthoz, G., and Schaefli, B.: Potential of Satellite and Reanalysis Evaporation Datasets for Hydrological Modelling under Various Model Calibration Strategies, Adv. Water Resour., 143, 103667, https://doi.org/10.1016/j.advwatres.2020.103667, 2020a.
Dembélé, M., Hrachowitz, M., Savenije, H. H. G., Mariéthoz, G., and Schaefli, B.: Improving the Predictive Skill of a Distributed Hydrological Model by Calibration on Spatial Patterns With Multiple Satellite Data Sets, Water Resour. Res., 56, e2019WR026085, https://doi.org/10.1029/2019wr026085, 2020b.
Dembélé, M., Schaefli, B., van de Giesen, N., and Mariéthoz, G.: Suitability of 17 gridded rainfall and temperature datasets for large-scale hydrological modelling in West Africa, Hydrol. Earth Syst. Sci., 24, 5379–5406, https://doi.org/10.5194/hess-24-5379-2020, 2020c.
Dembélé, M., Vrac, M., Ceperley, N., Zwart, S. J., Larsen, J., Dadson, S. J., Mariéthoz, G., and Schaefli, B.: Contrasting changes in hydrological processes of the Volta River basin under global warming, Hydrol. Earth Syst. Sci., 26, 1481–1506, https://doi.org/10.5194/hess-26-1481-2022, 2022.
Elagib, N. A., Al Zayed, I. S., Saad, S. A. G., Mahmood, M. I., Basheer, M., and Fink, A. H.: Debilitating floods in the Sahel are becoming frequent, J. Hydrol., 599, 126362, https://doi.org/10.1016/j.jhydrol.2021.126362, 2021.
ESGF: ESGF Node at DKRZ, ESGF [data set], https://esgf-data.dkrz.de, last access: 22 March 2020.
Fisher, N. I.: Statistical analysis of circular data, Cambridge University Press, 277 pp., https://doi.org/10.1017/CBO9780511564345, 1993.
Giuntoli, I., Vidal, J.-P., Prudhomme, C., and Hannah, D. M.: Future hydrological extremes: the uncertainty from multiple global climate and global hydrological models, Earth Syst. Dynam., 6, 267–285, https://doi.org/10.5194/esd-6-267-2015, 2015.
Gudmundsson, L., Boulange, J., Do, H. X., Gosling, S. N., Grillakis, M. G., Koutroulis, A. G., Leonard, M., Liu, J., Müller Schmied, H., and Papadimitriou, L.: Globally observed trends in mean and extreme river flow attributed to climate change, Science, 371, 1159–1162, https://doi.org/10.1126/science.aba3996, 2021.
Hanus, S., Hrachowitz, M., Zekollari, H., Schoups, G., Vizcaino, M., and Kaitna, R.: Future changes in annual, seasonal and monthly runoff signatures in contrasting Alpine catchments in Austria, Hydrol. Earth Syst. Sci., 25, 3429–3453, https://doi.org/10.5194/hess-25-3429-2021, 2021.
Kreibich, H., Van Loon, A. F., Schröter, K., Ward, P. J., Mazzoleni, M., Sairam, N., Abeshu, G. W., Agafonova, S., AghaKouchak, A., and Aksoy, H.: The challenge of unprecedented floods and droughts in risk management, Nature, 608, 80–86, https://doi.org/10.1038/s41586-022-04917-5, 2022.
Kumar, R., Samaniego, L., and Attinger, S.: Implications of distributed hydrologic model parameterization on water fluxes at multiple scales and locations, Water Resour. Res., 49, 360–379, https://doi.org/10.1029/2012wr012195, 2013.
Lane, R. A. and Kay, A. L.: Climate change impact on the magnitude and timing of hydrological extremes across Great Britain, Frontiers in Water, 3, 684982, https://doi.org/10.3389/frwa.2021.684982, 2021.
Lindersson, S., Brandimarte, L., Mård, J., and Di Baldassarre, G.: A review of freely accessible global datasets for the study of floods, droughts and their interactions with human societies, WIRES Water, 7, e1424, https://doi.org/10.1002/wat2.1424, 2020.
Mardia, K. V.: Statistics of directional data, Academic Press, London, 380 pp., ISBN 9781483218663, 1972.
Rameshwaran, P., Bell, V. A., Davies, H. N., and Kay, A. L.: How might climate change affect river flows across West Africa?, Climatic Change, 169, 21, https://doi.org/10.1007/s10584-021-03256-0, 2021.
Samaniego, L., Kumar, R., and Attinger, S.: Multiscale parameter regionalization of a grid-based hydrologic model at the mesoscale, Water Resour. Res., 46, W05523, https://doi.org/10.1029/2008wr007327, 2010.
Samaniego, L., Kumar, R., Thober, S., Rakovec, O., Schweppe, R., Schäfer, D., Schrön, M., Brenner, J., Demirel, C. M., Kaluza, M., Jing, M., Langenberg, B., and Attinger, S.: mesoscale Hydrologic Model (v5.9), Zenodo [code], https://doi.org/10.5281/zenodo.1299584, 2018.
Taylor, K. E., Stouffer, R. J., and Meehl, G. A.: An overview of CMIP5 and the experiment design, B. Am. Meteorol. Soc., 93, 485–498, https://doi.org/10.1175/BAMS-D-11-00094.1, 2012.
Van Vuuren, D. P., Edmonds, J., Kainuma, M., Riahi, K., Thomson, A., Hibbard, K., Hurtt, G. C., Kram, T., Krey, V., and Lamarque, J.-F.: The representative concentration pathways: an overview, Climatic change, 109, 5, https://doi.org/10.1007/s10584-011-0148-z, 2011.
Vetter, T., Reinhardt, J., Flörke, M., Van Griensven, A., Hattermann, F., Huang, S., Koch, H., Pechlivanidis, I. G., Plötner, S., and Seidou, O.: Evaluation of sources of uncertainty in projected hydrological changes under climate change in 12 large-scale river basins, Climatic Change, 141, 419–433, https://doi.org/10.1007/s10584-016-1794-y, 2017.
Villarini, G.: On the seasonality of flooding across the continental United States, Adv. Water Resour., 87, 80–91, https://doi.org/10.1016/j.advwatres.2015.11.009, 2016.
Vrac, M. and Thao, S.: R2D2 v2.0: accounting for temporal dependences in multivariate bias correction via analogue rank resampling, Geosci. Model Dev., 13, 5367–5387, https://doi.org/10.5194/gmd-13-5367-2020, 2020.
Ward, P. J., de Ruiter, M. C., Mård, J., Schröter, K., Van Loon, A., Veldkamp, T., von Uexkull, N., Wanders, N., AghaKouchak, A., and Arnbjerg-Nielsen, K.: The need to integrate flood and drought disaster risk reduction strategies, Water Security, 11, 100070, https://doi.org/10.1016/j.wasec.2020.100070, 2020.
Wilcox, C., Vischel, T., Panthou, G., Bodian, A., Blanchet, J., Descroix, L., Quantin, G., Cassé, C., Tanimoun, B., and Kone, S.: Trends in hydrological extremes in the Senegal and Niger Rivers, J. Hydrol., 566, 531–545, https://doi.org/10.1016/j.jhydrol.2018.07.063, 2018.
Yang, D., Yang, Y., and Xia, J.: Hydrological cycle and water resources in a changing world: A review, Geography and Sustainability, 2, 115–122, https://doi.org/10.1016/j.geosus.2021.05.003, 2021.
Yonaba, R., Biaou, A. C., Koïta, M., Tazen, F., Mounirou, L. A., Zouré, C. O., Queloz, P., Karambiri, H., and Yacouba, H.: A dynamic land use/land cover input helps in picturing the Sahelian paradox: Assessing variability and attribution of changes in surface runoff in a Sahelian watershed, Sci. Total Environ., 757, 143792, https://doi.org/10.1016/j.scitotenv.2020.143792, 2021.
Young, A. R., Round, C. E., and Gustard, A.: Spatial and temporal variations in the occurrence of low flow events in the UK, Hydrol. Earth Syst. Sci., 4, 35–45, https://doi.org/10.5194/hess-4-35-2000, 2000.