the Creative Commons Attribution 4.0 License.
the Creative Commons Attribution 4.0 License.
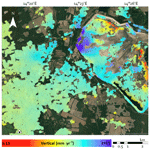
Introduction of Integrated Mining Impact Monitoring – i2Mon Development Project
Chia-Hsiang Yang
Andreas Müterthies
Monitoring of mining areas and associanted dam stability, has become increasingly important as the awareness of safety and environmental protection is rising. An appropriate monitoring scheme is necessitated to legally activate, reactivate, or terminate mining operations. The project Integrated Mining Impact Monitoring (i2Mon) aims to identify and analyze mining-induced impact, in particular its ground deformation. The monitoring system comprises terrestrial measurement and remote sensing: levelling, GPS, LiDAR scanning, UAV survey, and SAR interferometry. For interpretation and prediction, modelling will be used to simulate local displacements by different factors. The final goal is to launch an interactive GIS-based platform as an early warning and decision making system for mining industry. Currently, the project is proceeding from a preparatory phase. This paper focuses on spaceborne SAR interferometry, whereby we can cost-effectively monitor ground movement at millimeter level over a large area. We introduce the prototype of our InSAR monitoring system. The test result from Sentinel-1 images shows the surface movement during 2018 at a deactivating open-pit coal mine in Germany. We discuss the current status, ongoing works, planned test sites in Poland, and how we integrate data from different sensors and approaches.
- Article
(10892 KB) - Full-text XML
- BibTeX
- EndNote
For many countries, the mining industry accounts for an important component of their economy. In general, open-pit and underground mining causes surface deformation like ground subsidence. Monitoring this mining impact is increasingly important for the safety of human lives and properties. Recently, two catastrophic dam failures (Brumadinho dam disaster, 2019; Mariana dam disaster, 2019) in Brazil resulted in the loss of human lives (205 deaths and 122 missing persons) and countless property damages in 2015 and 2019. The investigation of the first disaster reveals there were signs of structural damage on the dam, which were earlier reported from on-site measurements. We believe such a disaster could be prevented by implementing a reliable monitoring system.
Generally, monitoring routines rely on in-situ surveys, which are considered reliable and accurate while expensive and time-consuming. A regular measurement campaign over an extensive area may not be cost effective. Alternatively, remote sensing based on spaceborne data offers an efficient and cost-effective alternative. This technique aims at frequent surveillance over large areas and provides comparable complements to in-situ measurement data. The information derived from both sides must be integrated into a united monitoring system.
The Integrated Mining Impact Monitoring (i2Mon) project (funded by the European Commission – Research Fund for Coal and Steel) intends to develop a mining monitoring system to the public. The data come from geodetic and geotechnical devices (e.g., levelling and GPS), laser scanning, UAV survey, and multi-spectral and radar satellites (e.g., Sentinel-1/-2 and TerraSAR-X/TanDEM-X). Movement phenomena are modelled to interpret the factors and physical processes at different spatiotemporal scales. The final goal is to launch an interactive GIS-based platform as an early warning and decision making system for mining industry.
This paper focuses on work package 2 in i2Mon. We are developing a monitoring system based on advanced InSAR processing of multi-temporal spaceborne SAR images. The persistent scatterer interferometry (PSI) (Crosetto et al., 2016; Ferretti et al., 2000, 2001; Hooper et al., 2004) and distributed scatterer interferometry (DSI) (Berardino et al., 2002; Ferretti et al., 2011; Lanari et al., 2007) approaches are used and adapted. These two approaches estimate surface movement across a broad area at millimeter accuracy. Spaceborne SAR delivers radar images, which are acquired regularly over large areas at a mission-oriented spatiotemporal resolution. Moreover, the active sensors are weather independent and have a day-and-night vision ability. This advantage makes SAR images free of cloud occlusion and always available for use. These characteristics make SAR suitable for long-term and global monitoring tasks.
In this context, we first illustrate our monitoring system in Sect. 2. Section 3 describes the activities that we have done so far and the ongoing plans. An initial DSI result is also illustrated here. Finally, we conclude this paper along with future works in Sect. 4.
The InSAR monitoring scheme (Fig. 1) operates as a self-contained chain starting from image acquisition to product delivery into the integrated system. Complementary data contain ground truth, a priori knowledge, and information from other techniques. Sentinel-1 and TerraSAR-X images are downloaded, processed, and stored at tailored intervals, e.g., every 6 and 11 d each, for use of InSAR processing. Requirements, strategies, and parameters are configured in preparation for a specific task. The InSAR processing – PSI and DSI is then implemented to generate initial results. They are analyzed to check if the requirements such as accuracy and coverage are met. If not, they are modified and evaluate again. Once the requirements are satisfied, the results are refined for different applications with respect to modelling, statistics, prediction, and so on. Subsequently, the relevant products under agreed formats are delivered to an integrated monitoring system. Here all data derived from different sensors and techniques are digested and cross-analyzed to produce decision elements. We are planning to build an interactive GIS-based platform as an early warning and decision making system for mining industry.
2.1 Spaceborne SAR Images
Civil spaceborne SAR sensors (Table 1) are currently in operation using X, C, and L bands. Three typical examples are TerraSAR-X, Sentinel-1, and PALSAR-2, respectively. Other operational and planned satellites include PAZ (X), COSMO-SkyMed (X), Radarsat-2 (C), RCM (C), SAOCOM (L), NISAR (L), and TanDEM-L (L) (https://directory.eoportal.org/web/eoportal/satellite-missions, last access: 20 February 2020).
Our monitoring system currently uses Sentinel-1 and TerraSAR-X. A standard Sentinel-1 image package covers an area around 300 km×200 km under TOPSAR acquisition mode. Such a large coverage enables synchronous monitoring of multiple mining areas. The meter-level resolution is sufficient to monitor mining impact. The shortest repeat cycle of 6 d empowers a weekly monitoring. Last but not least, Sentinel-1 images are free of charge for both scientific and commercial purposes. In comparison, although TerraSAR-X images are not offered for free, their results are characterized by high-resolution details and more precise movement estimate. If needed, we will turn to PALSAR-2, which is preferable if areas of interest are covered with dense vegetation.
2.2 InSAR Processing
PSI and DSI have their respective pros and cons and are adapted along with adequate strategies for scene monitoring. PSI detects and analyzes coherent target points from a SAR image sequence. These target points are characterized by stable, strong, and coherent signals reflected from a ground patch like a piece of a tailing dam. They are less affected by temporal decorrelation and contain mostly meaningful signals. The average velocity (up to sub-mm yr−1 accuracy) and time-series displacements are then evaluated for each point. Atmospheric noise are filtered out by applying a spatiotemporal filtering to the image series. However, target points are barely found from low- and moderate-coherence areas such as suburban scenes above underground mines. Similar to PSI, DSI also uses multi-temporal SAR images to monitor movement of target points on ground. The difference is that DSI is capable of providing meaningful results over low- and moderate-coherence areas; nevertheless, the accuracy (up to mm yr−1) is one order of magnitude lower than PSI.
2.3 Analysis
This step intends to validate InSAR results and examine whether they meet the requirements. We describe some important considerations in the following.
For the SAR analysis, coherence (Hanssen, 2001) is usually used to evaluate the quality of InSAR products. The higher a coherence of a point is, the higher precision of its result like movement estimate is expected. However, coherence is usually measured with a 0–1 range. Instead, our system converts wavelength and coherence to a metric form
where σM, λ, and γ are movement precision, wavelength, and coherence.
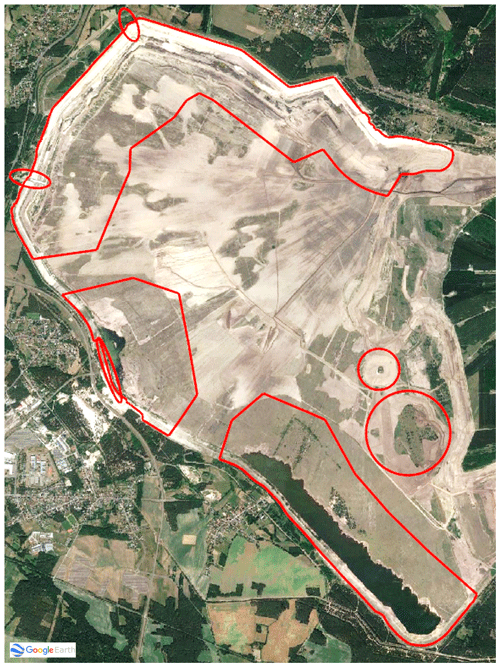
Figure 2Open-pit test mine (7.5 km×5.5 km) located at ( E, N) and owned by LEAG, Cottbus, Germany. Red enclosed area, priority area. Background image captured from © Google Earth.
For each monitoring instance, we double-check the consistency between InSAR results, which are derived from PSI and DSI using both ascending and descending acquisitions. If PSI and DSI results generate conflicting results, we must go thoroughly the procedure to identify and fix the inconsistencies.
The essences of objects must be considered to validate the associated InSAR results. For instance, the coherence over tailing areas is expected to be lower than the one over dam slopes. If this is not the case, the coherences might be either under- or overestimated, leading to false interpretation.
Last but not least, we also employ ground truth data in our analysis, including point-based data measured from GPS, levelling, and corner reflectors. For example, fixed points can be used as reference or check points. Their data formats, geographic locations, and temporal sampling are converted to be comparable to InSAR results.
2.4 Refinement
Our priority is to model the time-series movement of each target point. For this purpose, we involve spatiotemporal knowledge of mining activities and structural engineering in the model design. In-situ measurements like GPS and levelling are considered as complementary observations. Derived from modelling, the results provide movement estimates at different time scales. Given a proper model, the movement evolutions are resistant to noise and accurately reflective of mining activities. If needed, a temporal filtering can be applied to further suppress the noise before or after modelling. In addition, we also derive and analyze other physical quantities like velocity and acceleration based on statistics.
The project started in mid 2018 is about to finish its preparation phase. We have achieved some milestones so far. The work packages, techniques, website, data exchange, test sites and visits, system prototype and tests, sensors, and survey campaigns were discussed and reached a united agreement. The details are written in our first annual report submitted in mid 2019.
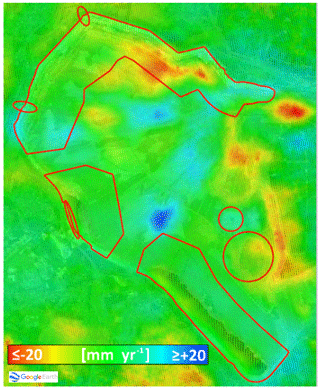
Figure 4DSI vertical velocity at open-pit test mine (14∘ E, N) (Fig. 2). Negative and positive, subsidence and heave. Red enclosed area, priority area (© Google Earth).
3.1 Open-pit Test Mine
Our test site is located next to Cottbus, Germany and is owned by LEAG (Fig. 2). It operated as a coal mine since 1981 and was closed at the end of 2015. Since 2016 the pit began to be converted into an artificial lake – Cottbuser Ostsee (https://www.leag.de/de/geschaeftsfelder/bergbau/cottbuser-ostsee/, last access: 20 February 2020). Monitoring of surface movement caused by previous mining is in particular crucial for this mission. Our monitoring focuses on the priority areas (red enclosed areas, Fig. 2), where water channeling, soil reinforcement, and embanking were planned. In March 2019 we visited and investigated the test site during our project meeting (Fig. 3). The information and data we collected are involved in our following works.
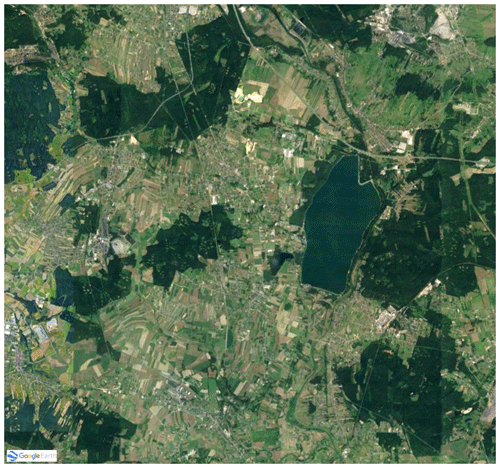
Figure 5Underground test site (15 km×15 km) owned by PGG, 60 km West of Krakow, Poland. Background image captured from Google Earth. Center at (19∘ E, 50∘ N) (© Google Earth).
Our first DSI result, derived from Sentinel-1 images, shows the overview of surface deformation in 2018 (Fig. 4). There are both subsidence and heave across the area. At first glance, one local engineer confirmed the overall deformation is reasonable and interpretable. We will later analyze our result in more detail and also compare it with the one derived from TerraSAR-X images.
3.2 Underground Test Mine
Currently, we are narrowing our test area down to a suburb (Fig. 5), where Polska Grupa Górnicza (PGG) is planning longwall coal mining activities in 2020. The tunnels will penetrate underneath existing buildings, where local ground subsidence is expected and could cause damages to these structures. We will provide InSAR results to analyze this impact. In July 2019 we visited some possible operation sites (Fig. 6) and discussed how to coordinate different working groups for the following activities. Our partner Airbus has scheduled TerraSAR-X to acquire high-resolution SAR images over this region. We must wait for the final confirmation from PGG to proceed with our monitoring tests.
This paper describes the initial prototype of our InSAR monitoring system, developed under the i2MON project, for mining areas. Using spaceborne SAR images, the system produces point-based motion data over an extensive area. Each point contains velocity, acceleration, movement series, and various statistics. The final goal is to launch an interactive GIS-based platform as an early warning system to the public. Under this scheme, the authority will be informed if there is a sign of subsidence beyond a threshold, which could cause serious building damages.
Our project just moved to next phase from preparation. We accomplished agreements partnered with LEAG and PGG to have an open-pit and an underground test mines, respectively. Our first DSI result, derived from Sentinel-1 images, shows the deformation velocity in 2018 on the open-pit test mine. Our local partner preliminarily considered the result meaningful and interpretable. More details will be released later, including a comparison with results derived from TerraSAR-X images.
Here come the future works among all partners. The acquisition of TerraSAR-X images is scheduled to make a stack over each test area. LEAG and PGG will provide supports and information like geologic maps and measurement data. To obtain ground truth, several in-situ campaigns are planned and scheduled with respect to GPS, levelling, UAV, laser scanning, corner reflectors, etc. Various kinds of models are being tested and designed. There are many works to be done until the next annual report around mid 2020.
Sentinel-1 images can be downloaded via Copernicus Open Access Hub https://scihub.copernicus.eu/ (last access: 20 February 2020: ESA, 2020). The access of other data are now only limited to the internal partners according to i2MON policy.
C-HY working for SAR remote sensing at EFTAS designed and programmed the approach. He also finished the first draft, including figures and tables. AM as the R&D coordinator at EFTAS supervised this study. He also proofread and approved the draft for submission.
The authors declare that they have no conflict of interest.
This article is part of the special issue “TISOLS: the Tenth International Symposium On Land Subsidence – living with subsidence”. It is a result of the Tenth International Symposium on Land Subsidence, Delft, the Netherlands, 17–21 May 2021.
Our work with i2MON is funded by the European Commission – Research Fund for Coal and Steel. Our partners include DMT, TU Delft, TU Bergakademie Freiber, UAS Mainz, Laserdata, Airbus, and IMG PAN. The Sentinel-1 and TerraSAR-X images are provided by Copernicus (funded by ESA) and Airbus, respectively. LEAG and PGG kindly grant our access to their mining sites for testing.
Berardino, P., Fornaro, G., Lanari, R., and Sansosti, E.: A new algorithm for surface deformation monitoring based on small baseline differential SAR interferograms, IEEE Trans. Geosci. Remote Sens., 40, 2375–2383, 2002.
Brumadinho dam disaster: available at: https://en.wikipedia.org/wiki/Brumadinho_dam_disaster, last access: 21 November, 2019.
ESA: Copernicus Open Access Hub, available at: https://scihub.copernicus.eu/dhus/#/home, last access: 20 February 2020.
Crosetto, M., Monserrat, O., Cuevas-González, M., Devanthéry, N., and Crippa, B.: Persistent scatterer interferometry: a review, ISPRS J. Photogramm. Remote Sens., 115, 78–89, 2016.
Ferretti, A., Prati, C., and Rocca, F.: Nonlinear subsidence rate estimation using permanent scatterers in differential SAR interferometry, IEEE Trans. Geosci. Remote Sens., 38, 2202–2212, 2000.
Ferretti, A., Prati, C., and Rocca, F.: Permanent scatterers in SAR interferometry, IEEE Trans. Geosci. Remote Sens., 39, 8–20, 2001.
Ferretti, A., Fumagalli, A., Novali, F., Prati, C., Rocca, F., and Rucci, A.: A new algorithm for processing interferometric data-stacks: SqueeSAR, IEEE Trans. Geosci. Remote Sens., 49, 3460–3470, 2011.
Hanssen, R. F.: Radar interferometry - data interpretation and error analysis, remote sensing and digital image processing, Springer, Dordrecht, the Netherlands, 2001.
Hooper, A., Zebker, H., Segall, P., and Kampes, B.: A new method for measuring deformation on volcanoes and other natural terrains using InSAR persistent scatterers, Geophys. Res. Lett., 31, L23611, https://doi.org/10.1029/2004GL021737, 2004.
Lanari, R., Casu, F., Manzo, M., Zeni, G., Berardino, P., Manunta, M., and Pepe, A.: An overview of the small baseline subset algorithm: a DInSAR technique for surface deformation analysis, Pure Appl. Geophys., 164, 637–661, 2007.
Mariana dam disaster: available at: https://en.wikipedia.org/wiki/Mariana_dam_disaster, last access: 21 November 2019.