the Creative Commons Attribution 4.0 License.
the Creative Commons Attribution 4.0 License.
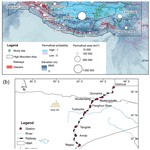
Assessment of permafrost-related hazards in China: based on Chinese literature
Prashant Baral
Miriam Jackson
High Mountain Asia (HMA) is undergoing unprecedented warming, affecting the cryosphere – including permafrost (frozen ground) – and leading to various hazards. However, understanding the prevalence, distribution, and dynamics of these hazards and how they respond to a changing climate is challenging. Permafrost is extensive in HMA, and China makes up a significant portion of this. The permafrost area in China is about 1.6×106 km2, 66 % of which is on the Qinghai–Tibet Plateau. However, most of the scientific literature concerning permafrost in China is published in Chinese and, hence, remains largely unnoticed by the non-Chinese-speaking scientific communities. In this article, we used a systematic review to evaluate the Chinese scientific literature on permafrost-related hazards and found that the studied areas are concentrated in certain areas, especially on the Qinghai–Tibet Engineering Corridor (QTEC). The increasing amount of literature on permafrost hazards reflects the increased impact of climate warming on infrastructure built on permafrost. Not only is permafrost affecting infrastructure; these anthropogenic disturbances themselves also have amplified the occurrence of hazards around settlements and infrastructure. The literature shows the strong relationship between latitude and elevation with permafrost thickness. The permafrost classification system and nomenclature used by Chinese scientists is different to that used elsewhere, which is a potential source of confusion and deserves attention.
- Article
(4226 KB) - Full-text XML
- BibTeX
- EndNote
High Mountain Asia (HMA) is undergoing unprecedented warming (Yao et al., 2019; Hock et al., 2019), causing considerable changes to the cryosphere (Bolch et al., 2019), including to the permafrost in high mountains and on mountain plateaus (Li et al., 2022). China constitutes a large portion of HMA, including several high mountain ranges, with a significant distribution of glaciers and permafrost; transboundary river basin systems; and the highest plateau in the world, the Qinghai–Tibetan Plateau (QTP). In China, about 1.6×106 km2 is considered to be permafrost; about 66 % of this in the QTP, 15 % is in northeastern China, and 19 % is in the mountains of the western and central parts of the country (Ran et al., 2012).
Permafrost is commonly defined as frozen ground at or below 0 °C for at least 2 consecutive years (Dobinski, 2011). It is a thermal phenomenon (Gruber Stephan and Haeberli, 2009) and is often identified using ground surface temperature measurements or visible indicators such as rock glaciers or other slow-moving bodies of rock and ice that exist in permafrost environments. Globally, permafrost areas are responding to higher air temperatures; with higher ground temperatures and the thickening of the active layer or the soil portion above permafrost that undergoes seasonal freezing and thawing (Dobiński, 2020), permafrost areas are thawing, warming, degrading, and disappearing, consequently increasing the risks of rockfalls, debris flows, thaw slumps, and permafrost-dammed lake outbursts (Ding et al., 2021; Biskaborn et al., 2019). Permafrost warming and degradation influence not only cryosphere-related landforms but also fluvial landforms and processes by controlling the surface runoff, by eroding sediments, and through the development of thermo-erosional gullies (Tananaev and Lotsari, 2022). The degradation of permafrost holds particular significance in mountainous regions due to its profound impact on slope stability. Consequently, this process modifies the geophysical attributes of mountain slopes, diminishing cohesive forces and elevating hydraulic conductivity (Patton et al., 2019). Water deposits affecting hydrothermal exchanges, fluvio-thermal erosion due to surface runoff, and swelling and subsidence of frozen grounds due to frost heave and thaw settlements strongly affect infrastructure built on thawing-permafrost grounds.
Understanding the probability and dynamics of potential risks due to permafrost thaw are vital for hazard reduction and safeguarding critical infrastructure (Harris et al., 2009). The QTP holds significant importance in permafrost research, primarily because it houses critical infrastructures like the Qinghai–Tibet railway and Qinghai–Tibet highway. Within China, numerous specialized research programs are actively studying the interplay between changing permafrost conditions and their impact on infrastructure, as well as the reciprocal effects of infrastructure on permafrost (Wu et al., 2010; Cheng and Jin, 2013; Ran et al., 2022). There is extensive Chinese literature and a comprehensive database, such as the China National Knowledge Infrastructure, containing an extensive compilation of permafrost-related publications and summaries. This wealth of knowledge is often overlooked and excluded in systematic assessments and reviews of permafrost changes in High Mountain Asia (Baral et al., 2023). Our systematic review tries to advance the scientific knowledge related to climate change impacts on permafrost dynamics and the occurrence of hazards related to thawing permafrost discussed in Chinese literature by making it more accessible to the English-speaking scientific community. This study attempts to answer the following questions to reveal to a wider audience the information stored in the Chinese literature database:
-
According to Chinese scientific literature, what are the major hazards associated with changing permafrost in China and how are they distributed across Chinese territories within HMA?
-
What are the major particularities in the Chinese literature that are different from the English literature?
-
Are specific trends related to the occurrence of hazards visible?
-
What future concerns are related to infrastructure construction and maintenance in the context of global warming and changes in permafrost in China?
Our results are totally based on the outcome of the systematic review of the Chinese literature, while the Introduction and Discussion sections are largely based on the information about permafrost-related hazards in HMA, mainly in China, in the English literature. In some cases, we mention the Hindu Kush Himalaya (HKH), referring to the region ranging from 15.95 to 39.31° N and 60.85 to 105.04° E, which includes the high-elevation regions in China, Bhutan, India, Pakistan, Nepal, Afghanistan, Bangladesh, and Myanmar (Bajracharya and Shrestha, 2011).
2.1 Study area
To understand permafrost and its related hazards, our research is centered in the ecologically significant region of High Mountain Asia. This vast area encompasses some of the world's highest and most imposing mountain ranges, including the Himalayas, Karakoram, Hindu Kush, and Pamir. HMA is characterized by its extreme elevations, low temperatures, and rugged terrain, making it a prime location for the study of permafrost dynamics and associated risks.
Most of the study sites were located on the Qinghai–Tibet Plateau, an integral part of the HMA region, often referred to as the “Third Pole” due to its immense ice reserves. The QTP is the highest and largest plateau on Earth and is situated in western China and parts of Tibet. Its unique and remote landscape presents an ideal setting for the study of permafrost due to its extensive permafrost coverage and sensitivity to climate change. The Qinghai–Tibet Engineering Corridor (QTEC) encompasses a network of critical infrastructure projects, including highways, railways, and energy developments, spanning the vast and challenging terrain of the QTP. This corridor presents a unique opportunity to study the interaction between human activities and the delicate permafrost environment.
2.2 Systematic literature review method
This study built on the principles of the systematic review approach to assess the Chinese-language literature on permafrost hazards in High Mountain Asia and included the influence of structures on permafrost (James et al., 2016; Haddaway and Macura, 2018). In sustaining the replicability and transparency of systematic reviews, we adopted the RepOrting standards for Systematic Evidence Syntheses (ROSES) framework for ensuring evidence synthesis; this framework presents a protocol for searching, screening, and synthesis (Fig. S1 in Pan et al., 2024, https://doi.org/10.5281/zenodo.12157179). The following relevant indicators were included in the compilation: site information (coordinate, temperature), permafrost (thickness, active-layer thickness), hazard category, and infrastructure (type, area, length).
3.1 Distribution of study areas
The place with the most hazard records was the QTEC (Fig. 2). Obviously, Chinese literature paid more attention to this area. A total of 10 distinctive hazards took place in this engineering corridor, including collapse, thaw slumps, frost weathering, frost heaving, thermokarst lake, thaw settlement, damage to infrastructure, ice wedge, debris flow, and landslides (e.g., Luo et al., 2014; Ma et al., 2012). Qilian Shan mainly suffered from thaw slumps, frost weathering, frost heaving, thaw settlements, ice wedges, debris flows, and landslides (e.g., Mu, 2021; Pang et al., 2019; Shang, 2018). Western Kunlun Shan experienced thaw slumps, frost heaving, thaw settlements, salivary ice, and desertification (e.g., Ma et al., 2004; Zhu, 2017). Tien Shan, which is outside the QTP but located in HMA, was found to be affected by thaw slump, frost heaving, ice wedge, and landslide (e.g., Ci et al., 2006). Besides this, thermokarst lakes, ice wedges, debris flows, and landslides were exclusively recorded in the east of the QTP.
All field sites were clustered into four zones around the QTEC, Qilian Shan, western Kunlun Shan, and Tien Shan. The elevation gradient was between 2800 to 5886 m. The latitude and longitude ranges of these sites were 30.5 to 44.0° N and 78.3 to 105.9° E (Fig. 3a). Field-based research sites in the mountains are distributed within the wide-ranging elevation from 2800 m to almost 6000 m a.s.l., while research sites in the river basins spread from around 3000 to 5200 m a.s.l. (Fig. 3b). Consequently, mean annual air temperature (MAAT hereafter) and mean annual ground temperature (MAGT hereafter) values recorded at the study sites in the mountains have a wider range of values compared to those of the study sites in the river basins. The distribution of MAAT in the river basins varies from −6.24 to −0.5 °C, while in the mountains, MAAT ranges from −9 to −4.3 °C. MAGT has a very narrow range in the basins (−1.75 to −0.5 °C) compared to in the mountains (−4.25 to 1.1 °C).
Active-layer thickness varies from 1 to 6 m for MAGT ranging from −5 to slightly more than 1 °C; study sites record MAAT between −8 and 1 °C (Fig. S3 in Pan et al., 2024). The thickness of permafrost reported for multiple field sites ranges between 0 to 200 m. In locations where MAGT ranges from −2 to 0 °C, permafrost thickness is usually below 50 m. Permafrost thickness is mostly reported for sites with MAAT from −6 to −3 °C.
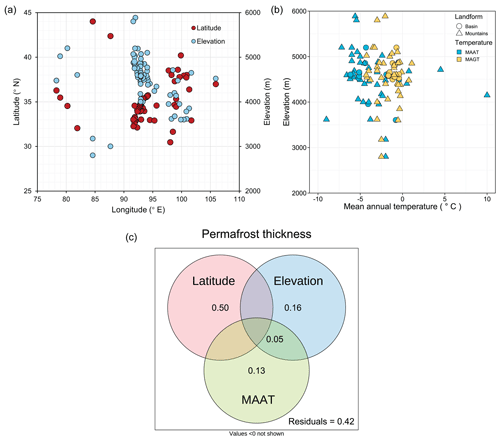
Figure 3Coordinates and elevations given in publications (a). Elevation-dependent mean annual air and ground temperature changes over HMA (b). Variation partition analysis (VPA) revealing the impacts of latitude, elevation, and mean annual air temperature on permafrost thickness (c). The values given in the non-overlapping parts of each circle give the weight of each factor. The value given in the overlapping circles gives the explanation of the variation between two factors.
3.2 Influence of different parameters
Coordinates, topography, and temperature all show a correlation with the variance in permafrost thickness (Wang et al., 1979; Liu, 2008; Yin et al., 2014). In particular, latitude, elevation, and mean annual air temperature all independently explained the variance in permafrost thickness. The best model for understanding the controls on permafrost thickness indicated that latitude controlled 50 % of the variation, and elevation explained 16 % independently of the 13 % explained by the MAAT. Elevation and MAAT shared a small amount of variance in terms of permafrost thickness (5 %), confirming the MAAT specificity of elevation (Fig. 3c). The permafrost on the Qinghai–Tibet Plateau was influenced by elevation and followed the latitudinal zonation pattern (Zhou et al., 2000). The law of decreasing the lower limit of permafrost with increasing latitude was quite evident. On average, for every 1° increase in latitude, the permafrost lower limit decreased by 80–100 m, and the annual average ground temperature decreased by 0.9–1 °C (Wang et al., 1979; Bian et al., 2011). There was also a solid vertical zonation pattern of increasing permafrost thickness with higher elevation. For approximately every 100 m increase in elevation, the annual average ground temperature decreased by 0.5–0.6 °C, and the permafrost thickness increased by 15–20 m (Bian et al., 2011).
4.1 Permafrost in English and Chinese scientific literature
In China, permafrost has been systematically investigated since the 1950s (Zhang, 2005). Development of permafrost science in China has been closely associated with the infrastructure and economic development; therefore, there are dedicated institutions in China with decades of intensive research. Permafrost research in China is based on standard definitions and classifications (Tables S1, S2, and S3 in Pan et al., 2024) commonly accepted by Chinese institutions and researchers. These standard definitions and classifications do not always match with the contemporary definitions and classifications accepted by the English scientific community (Ran et al., 2012). For example, the International Permafrost Association defines the percentage of permafrost for continuous, discontinuous, sporadic, and isolated patches to be 90 %–100 %, 50 %–90 %, 10 %–50 %, and 0 %–10 %, respectively, while some of the Chinese literature uses continuous, discontinuous, and island permafrost with >65 %, 30 %–65 %, and 5 %–30 %, respectively (Qinglai, 2007). Similarly, although the classification of permafrost based on ice content is well established in the English literature, the Chinese literature uses the classification based on the ice content criterion (Table S1 in Pan et al., 2024), termed the permafrost engineering classification. Additionally, there are several Chinese terms that are commonly used in Chinese scientific literature but that are not common or are not used at all in English scientific literature. Some examples of such are Chinese terms whose literary translations in English are “salivary ice”, “extruded ice”, and “ice mantle” (e.g., Liu et al., 2003; Liu, 2008; Ma et al., 2004).
4.2 Permafrost hazards
Thermokarst terrains, such as retrogressive thaw slumps, are increasing freeze–thaw erosions and modifying landscapes on the Qinghai–Tibet Plateau (QTP). Permafrost warming has extensively increased permafrost collapse events across the Qinghai–Tibetan Plateau, affecting pedological characteristics and soil microbial communities (Hu and Yang, 2023).
Permafrost degradation has some major implications for infrastructure: damages to embankments, failure of bridges, and longitudinal cracks along highways (Hjort et al., 2022). Large-area coverage of permafrost (about two-fifths of the total area) on the QTP includes large sections of highways and several thousands or kilometers of railway lines; notably, from 1991 to 2011, almost USD 1 billion were spent on the maintenance of QTP. The cost for mitigation of damages to ensure the smooth functioning of highways and railway networks in China could reach up to several billions of US dollars between 2051–2100 (Hjort et al., 2022).
Regular field-based monitoring of meteorological variables using automated weather stations, soil moisture and temperature measurements where infrastructure foundations are located, regular surveys of the ground subsidence, stability and support assessments, and effective implementation of early-warning systems are critical for infrastructure located in thawing-permafrost regions (Wang et al., 2019).
Changes in ground ice strongly determine risks for communities on the QTP. Simulations suggest that permafrost area on the QTP decreased by about 0.25×106 km2, while ground temperatures increased by 0.25 °C per decade between 1981 and 2010 (Xu et al., 2017). Freeze–thaw hazards and thaw settlements due to ground ice changes will have impacts on existing and planned infrastructure and so will have subsequent economic implications. Elevation-dependent temperature response will considerably increase the active-layer thickness and decrease permafrost areas on the Tibetan Plateau (Wang et al., 2022), increasing the risks of geohazards.
A more detailed discussion about permafrost hazards, adaptation to these hazards, and future projections can be found in Pan et al. (2024) (Sup_Assessment of permafrost-related hazards in China based on Chinese literature.docx)
The volume of scientific literature is increasing; the QTEC is an obvious geographical preference for conducting measurements and assessments of permafrost-related hazards. Studies and measurements are concentrated around a few hotspot areas, while extensive areas in China remain unexamined.
Projections of hazards based on complex modeling approaches using projected socioeconomic global changes are limited. Expanding measurements and research will be needed to provide a comprehensive picture of the dynamics of changing permafrost and potential hazards.
Increasing warming is likely to invite climate extremes and increase the frequency of cascading and compounding events linked to permafrost thawing. Human populations and infrastructures exposed to permafrost hazards will increase as a consequence of increasing human activities in changing permafrost areas.
Finally, warming permafrost has direct and indirect biophysical impacts, such as landscape changes, hydrological variations, and greenhouse gas releases, as well as socio-economic impacts such as damage to human settlements and infrastructure, which, in turn, can constrain permafrost distribution and dynamics and the occurrence of permafrost hazards.
The dataset is available at https://doi.org/10.5281/zenodo.12157179 (Pan et al., 2024).
SP collected and analyzed the data, prepared the figures, and wrote the paper. PB contributed to the paper and prepared the map of the study sites. MJ assisted with subsequent revisions.
The contact author has declared that none of the authors has any competing interests.
Publisher's note: Copernicus Publications remains neutral with regard to jurisdictional claims made in the text, published maps, institutional affiliations, or any other geographical representation in this paper. While Copernicus Publications makes every effort to include appropriate place names, the final responsibility lies with the authors.
This article is part of the special issue “Mountain Hydrology and Cryosphere”. It is a result of the International Conference on Mountain Hydrology and Cryosphere, Kathmandu and Dhulikhel, Nepal, 9–10 November 2023.
Completion of the manuscript was supported by core funds of ICIMOD contributed to by the governments of Afghanistan, Australia, Austria, Bangladesh, Bhutan, China, India, Myanmar, Nepal, Norway, Pakistan, Switzerland, and the United Kingdom.
Bajracharya, S. R. and Shrestha, B.: The status of glaciers in the Hindu Kush-Himalayan region, International Centre for Integrated Mountain Development (ICIMOD), https://doi.org/10.53055/ICIMOD.551, 2011.
Baral, P., Allen, S., Steiner, J. F., Gurung, T. R., and McDowell, G.: Climate change impacts and adaptation to permafrost change in High Mountain Asia: a comprehensive review, Environ. Res. Lett., 18, 093005, https://doi.org/10.1088/1748-9326/acf1b4, 2023.
Bian, J., Kou, L., and Zhan, B.: The classification of frozen hazards and protecting approach of permafrost region In Tibetan Plateau permafrost region, The Chinese Journal of Geological Hazard and Control, 22, 129–133, 2011.
Biskaborn, B. K., Smith, S. L., Noetzli, J., Matthes, H., Vieira, G., Streletskiy, D. A., Schoeneich, P., Romanovsky, V. E., Lewkowicz, A. G., Abramov, A., Allard, M., Boike, J., Cable, W. L., Christiansen, H. H., Delaloye, R., Diekmann, B., Drozdov, D., Etzelmüller, B., Grosse, G., Guglielmin, M., Ingeman-Nielsen, T., Isaksen, K., Ishikawa, M., Johansson, M., Johannsson, H., Joo, A., Kaverin, D., Kholodov, A., Konstantinov, P., Kröger, T., Lambiel, C., Lanckman, J. P., Luo, D., Malkova, G., Meiklejohn, I., Moskalenko, N., Oliva, M., Phillips, M., Ramos, M., Sannel, A. B. K., Sergeev, D., Seybold, C., Skryabin, P., Vasiliev, A., Wu, Q., Yoshikawa, K., Zheleznyak, M., and Lantuit, H.: Permafrost is warming at a global scale, Nat. Commun., 10, 264, https://doi.org/10.1038/s41467-018-08240-4, 2019.
Bolch, T., Shea, J. M., Liu, S., Azam, F. M., Gao, Y., Gruber, S., Immerzeel, W. W., Kulkarni, A., Li, H., Tahir, A. A., Zhang, G., and Zhang, Y.: Status and Change of the Cryosphere in the Extended Hindu Kush Himalaya Region, in: The Hindu Kush Himalaya Assessment, edited by: Wester, P., Mishra, A., Mukherji, A., and Shrestha, A., Springer, Cham, https://doi.org/10.1007/978-3-319-92288-1_7, 2019.
Cheng, G. and Jin, H.: Permafrost and groundwater on the Qinghai-Tibet Plateau and in northeast China, Hydrogeol. J., 21, 5–23, https://doi.org/10.1007/s10040-012-0927-2, 2013.
Ci, J., Zhang, Y., Li, C., and Hou, X.: Characteristics and hazards of permafrost from Dushanzi to Qiaoerma in the Tianshan range, Xinjiang, Xinjiang Geology, 24, 71–74, 2006.
Ding, Y., Mu, C., Wu, T., Hu, G., Zou, D., Wang, D., Li, W., and Wu, X.: Increasing cryospheric hazards in a warming climate, 213, 103500, https://doi.org/10.1016/j.earscirev.2020.103500, 2021.
Dobinski, W.: Permafrost, Earth Sci. Rev., 108, 158–169, https://doi.org/10.1016/j.earscirev.2011.06.007, 2011.
Dobiński, W.: Permafrost active layer, Earth Sci. Rev., 208, 103301, https://doi.org/10.1016/j.earscirev.2020.103301, 2020.
Gruber, S. and Haeberli, W.: Mountain Permafrost, in: Permafrost Soils, edited by: Margesin, R., Springer Berlin Heidelberg, Berlin, Heidelberg, 33–44, https://doi.org/10.1007/978-3-540-69371-0_3, 2009.
Haddaway, N. R. and Macura, B.: The role of reporting standards in producing robust literature reviews, Nat. Clim. Change, 8, 444–447, https://doi.org/10.1038/s41558-018-0180-3, 2018.
Harris, C., Arenson, L. U., Christiansen, H. H., Etzelmüller, B., Frauenfelder, R., Gruber, S., Haeberli, W., Hauck, C., Hölzle, M., Humlum, O., Isaksen, K., Kääb, A., Kern-Lütschg, M. A., Lehning, M., Matsuoka, N., Murton, J. B., Nötzli, J., Phillips, M., Ross, N., Seppälä, M., Springman, S. M., and Vonder Mühll, D.: Permafrost and climate in Europe: Monitoring and modelling thermal, geomorphological and geotechnical responses, Earth-Sci. Rev., 92, https://doi.org/10.1016/j.earscirev.2008.12.002, 2009.
Hock, R., Rasul, G., Adler, C., Cáceres, B., Gruber, S., Hirabayashi, Y., Jackson, M., Kääb, A., Kang, S., Kutuzov, S., Milner, A., Molau, U., Morin, S., Orlove, B., and Steltzer, H.: High Mountain Areas, in: IPCC Special Report on the Ocean and Cryosphere in a Changing Climate, edited by: Pörtner, H.-O., Roberts, D. C., Masson-Delmotte, V., Zhai, P., Tignor, M., Poloczanska, E., Mintenbeck, K., Alegría, A., Nicolai, M., Okem, A., Petzold, J., Rama, B., and Weyer, N. M., Cambridge University Press, Cambridge, UK and New York, NY, USA, 131–202, https://doi.org/10.1017/9781009157964.004, 2019.
Hjort, J., Streletskiy, D., Doré, G., Wu, Q., Bjella, K., and Luoto, M.: Impacts of permafrost degradation on infrastructure, Nat. Rev. Earth Environ., 3, 24–38, https://doi.org/10.1038/s43017-021-00247-8, 2022.
Hu, X. and Yang, Z.-G.: Changes in Soil Microbial Community during Permafrost Collapse Process on the Northeast Qinghai-Tibetan Plateau, China, J. Soil Sci. Plant Nutr., 23, 4157–4167, https://doi.org/10.1007/s42729-023-01332-1, 2023.
James, K. L., Randall, N. P., and Haddaway, N. R.: A methodology for systematic mapping in environmental sciences, Environ. Evid., 5, 7, https://doi.org/10.1186/s13750-016-0059-6, 2016.
Li, D., Lu, X., Walling, D. E., Zhang, T., Steiner, J. F., Wasson, R. J., Harrison, S., Nepal, S., Nie, Y., Immerzeel, W. W., Shugar, D. H., Koppes, M., Lane, S., Zeng, Z., Sun, X., Yegorov, A., and Bolch, T.: High Mountain Asia hydropower systems threatened by climate-driven landscape instability, Nat. Geosci., 15, 520–530, https://doi.org/10.1038/s41561-022-00953-y, 2022.
Liu, C., Li, T., and Lin, Y.: Study on environment geology impact assessment for highway rebuilding in westward altiplano region, Environmental Protection in Transportation, 24, 8–11, 2003.
Liu, Z.: Distribution characteristics of permafrost development along Qinghai-Tibet Railway, Railway Investigation and Surveying, 24, 78–82, 2008.
Luo, J., Niu, F., Lin, Z., Liu, M., and Yin, G.: Development of thawing hazards and thermal influence on permafrost along Qinghai-Tibet enginering corridor, Journal of Engineering Geology, 22, 326–333, 2014.
Ma, D., Zhang, J., Wang, M., Zhao, J., and Gao, W.: Characteristics and hazards of permafrost along the Xinjiang-Tibetan Highway, Xinjiang, J. Mt. Sci., 22, 554–561, 2004.
Ma, W., Niu, F., and Mu, Y.: Basic research on the major permafrost projects in the Qinghai-Tibet plateau, Adv. Earth Sci., 27, 185–191, 2012.
Mu, M.: Characteristics and impact factors of carbon release from thaw slump landforms in Qilian, Master dissertation, Lanzhou University, Lanzhou, https://ir.lzu.edu.cn/handle/262010/460095 (last access: 6 August 2024), 2021.
Pan, S., Baral, P., and Jackson, M.: Sup_Assessment of permafrost related hazards in China based on Chinese literature, Zenodo [data set], https://doi.org/10.5281/zenodo.12157179, 2024.
Pang, W., Chang, G., Gou, H., and Wang, Y.: Characteristics of the permafrost from Qilian to Mole of the Highway 204 in Qinghai Province, J. Glaciol. Geocryol., 41, 93–99, 2019.
Patton, A. I., Rathburn, S. L., and Capps, D. M.: Landslide response to climate change in permafrost regions, Geomorphology, 340, 116–128, https://doi.org/10.1016/j.geomorph.2019.04.029, 2019.
Qinglai, C.: Engineering geologial research on the permafrost in high lattitudes area and its impact on pipeline construction, PhD dissertation, Chinese Academy of Geological Sciences, Beijing, https://kns.cnki.net/KCMS/detail/detail.aspx?dbname=CDFD0911&filename=2007213368.nh (last access: 6 August 2024), 2007.
Ran, Y., Li, X., Cheng, G., Zhang, T., Wu, Q., Jin, H., and Jin, R.: Distribution of Permafrost in China: An Overview of Existing Permafrost Maps, Permafrost Periglac., 23, 322–333, https://doi.org/10.1002/ppp.1756, 2012.
Ran, Y., Cheng, G., Dong, Y., Hjort, J., Lovecraft, A. L., Kang, S., Tan, M., and Li, X.: Permafrost degradation increases risk and large future costs of infrastructure on the Third Pole, Commun. Earth Environ., 3, 328, https://doi.org/10.1038/s43247-022-00568-6, 2022.
Shang, J.: Study on change of thaw slump based on remote sensing Over the Eboling Ridge in permafrost region of Qilian Mountains, Master dissertation, Lanzhou University, Lanzhou, https://kns.cnki.net/KCMS/detail/detail.aspx?dbname=CMFD201802&filename=1018979558.nh (last access: 6 August 2024), 2018.
Tananaev, N. and Lotsari, E.: Defrosting northern catchments: Fluvial effects of permafrost degradation, 228, 103996, https://doi.org/10.1016/j.earscirev.2022.103996, 2022.
Wang, F., Li, G., Ma, W., Wu, Q., Serban, M., Vera, S., Alexandr, F., Jiang, N., and Wang, B.: Pipeline–permafrost interaction monitoring system along the China–Russia crude oil pipeline, Eng. Geol., 254, 113–125, https://doi.org/10.1016/j.enggeo.2019.03.013, 2019.
Wang, J., Wang, S., and Qiu, G.: Permafrost along the Qinghai-Xizang Highway, Acta Geogr. Sin., 34, 18–32, 1979.
Wang, X., Ran, Y., Pang, G., Chen, D., Su, B., Chen, R., Li, X., Chen, H. W., Yang, M., Gou, X., Jorgenson, M. T., Aalto, J., Li, R., Peng, X., Wu, T., Clow, G. D., Wan, G., Wu, X., and Luo, D.: Contrasting characteristics, changes, and linkages of permafrost between the Arctic and the Third Pole, Earth-Sci. Rev., 230, 104042, https://doi.org/10.1016/j.earscirev.2022.104042, 2022.
Wu, Q., Zhang, T., and Liu, Y.: Permafrost temperatures and thickness on the Qinghai-Tibet Plateau, Global Planet. Change, 72, 32–38, https://doi.org/10.1016/j.gloplacha.2010.03.001, 2010.
Xu, X., Zhang, Z., and Wu, Q.: Simulation of permafrost changes on the Qinghai–Tibet Plateau, China, over the past three decades, Int. J. Digit. Earth, 10, 522–538, https://doi.org/10.1080/17538947.2016.1237571, 2017.
Yao, T., Xue, Y., Chen, D., Chen, F., Thompson, L., Cui, P., Koike, T., Lau, W. K.-M., Lettenmaier, D., Mosbrugger, V., Zhang, R., Xu, B., Dozier, J., Gillespie, T., Gu, Y., Kang, S., Piao, S., Sugimoto, S., Ueno, K., Wang, L., Wang, W., Zhang, F., Sheng, Y., Guo, W., Ailikun, Yang, X., Ma, Y., Shen, S. S. P., Su, Z., Chen, F., Liang, S., Liu, Y., Singh, V. P., Yang, K., Yang, D., Zhao, X., Qian, Y., Zhang, Y., and Li, Q.: Recent Third Pole's Rapid Warming Accompanies Cryospheric Melt and Water Cycle Intensification and Interactions between Monsoon and Environment: Multidisciplinary Approach with Observations, Modeling, and Analysis, B. Am. Meteor. Soc., 100, 423–444, https://doi.org/10.1175/BAMS-D-17-0057.1, 2019.
Yin, G., Niu, F., Lin, Z., Luo, J., Liu, M., and Li, A.: The distribution characteristics of permafrost along the Qinghai-Tibet Railway and their response to environmental change, J. Glaciol. Geocryol., 36, 772–781, 2014.
Zhang, T.: Historical overview of permafrost studies in China, Phys. Geogr., 26, 279–298, https://doi.org/10.2747/0272-3646.26.4.279, 2005.
Zhou, Y., Guo, D., Qiu, G., Cheng, G., and Li, S.: Geocryology in China, 1st edn., Science Press, Beijing, ISBN 9787030082855, 2000.
Zhu, F.: Mechanism of frost heave and thaw settlement of subgrade and stability evaluation of subgrade in alpine permafrost regions, Master dissertation, Chang'an University, Xi'an, https://kns.cnki.net/KCMS/detail/detail.aspx?dbname=CMFD201801&filename=1017870452.nh (last access: 6 August 2024), 2017.