the Creative Commons Attribution 4.0 License.
the Creative Commons Attribution 4.0 License.
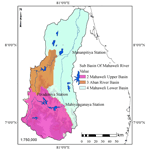
Significance of Having Integrated Water Resource Plan in a Complex Watershed System for Better Water Management during Covid Pandemic: The Case of Mahaweli River Basin
Roshan Indika Jayasinghe
Mohamad Rasmy
Toshio Koike
Water scarcity and disasters, particularly floods and droughts, are the most devastating disasters in Sri Lanka, causing severe damage to agriculture, lives and property. This condition has been worsen further during the Covid19, due to isolation and travel restrictions. Collaboration and effective watershed management among the stakeholders is one of the key solutions for effective operation, but watershed management is more complex and complicated due to its physical processes, and conflicts among stakeholders. This study attends to attract stakeholders and develop Decision Making System by integrating complex hydrological model with dam operation and diversion, based on topography, rainfall, evaporation with bias-corrected satellite data. Finally, we developed an Integrated System for decision makers for effective water resource management in River basins. The proposed approach was applied to the complex Mahaweli watershed in Sri Lanka, to address prevailing issues.
- Article
(7184 KB) - Full-text XML
- BibTeX
- EndNote
A watershed is area, which drained the each drop of water to single outlet that demarcated topographically. This watershed can be vary in size from small to thousands of square kilometres. Among these watersheds, huge type of natural disasters are common, such as flood, debris flow, landslide, and drought. Understanding and managing the watershed play the crucial role to minimize the damage and vulnerability. However, understanding and managing watershed is complex due to its hydrological processes. The watersheds are not simple or linear systems due to several reasons, e.g., spatial and temporal changes of precipitation, evaporation, infiltration, snowmelt process, water storage and diversion, vegetation type and its penology, land-use and soil characteristics, topography, and river morphology. Particularly, human-made changes such as water storage and their usage for power generation, irrigation water supply, diversion to other basins, and drinking water requirement, make the hydrological processes complicated. In addition, the frequent confliction between the users and stakeholders, become more complex, while managing available resources during the wet and dry period.
Focusing the Mahaweli river basin will get the overall model for the complex watershed management. Because, this river basin is largest in Sri Lanka and flows through wet, intermediate and dry zone. Throughout the journey, it meet different activities, such as flood control and storages in reservoirs, trans-basin diversion, and cultivation more than 165 000 ha of agricultural lands, and hydropower generation, which produce 25 %–30 % of country demand (Annual Reports of Mahaweli Authority of Sri Lanka, 2018). Sharing these activities by multiple organizations will causes the confliction among the stakeholders. During the Covid pandemic, it further worsen, due to isolation of people and limited working capacity in the organizations. Developing integrated water resource plan is essential to manage the available resources and disasters within such complex watershed effectively.
Dynamic system approach is one of the best method to overcome the complexity of the watershed (Khan et al., 2007). However, it will not perform well in extreme events and long-term processers due to systems uncertainties and multipurpose behaviours. There are hydrological models developed to understand the hydrological behaviour, but those are simpler and difficult to apply for complex behaviour. Due to this problem science-based system approach (Xin et al., 2018) combined with the complex system for Integrated Water Resources Management (IWRM), by considering the complicated physical activities, which limited understanding of physical processes, and limited facilities to introduce human-made activities. During the our study, Rainfall-Runoff Inundation Model (Sayama, 2013) selected to understand the hydrological processes in the basin, due to its flexibility and diffusion wave approximation of the stream, and incorporate the human-made changes to this model and developed Complex System Approach Model (CSAModel).
This CSAModel applied to complex Mahaweli river basin to identify the capabilities by using its physical behaviours. Then reservoirs and diversions introduced to complete the complex behaviour (Sajjad et al., 2000). Then, dam optimization technics were introduced to calculate the hydropower generation, water distribution, and flood management. The outcome shows the realistic results to stakeholders to manage the system even in the pandemic period as one intergraded system.
2.1 Complex system approach
The approach developed to understand the complexity of the watershed, by dividing the watershed to different sections, which hydrological system, geological system, and man-made system, for better performance. The final combined product used for its applications of combined complex hydrological model, to understand the present condition, and policy development for effective basin management.
2.1.1 Hydrological system
Hydrological system include the basic hydrological model considering topography, and spatial and temporal variation of rainfall, infiltration, and evapotranspiration.
2.1.2 Geological system
Geological behaviour in the watershed considered with sub division of the main tributaries. This helped to include the different behaviours of the watershed.
2.1.3 Man-made system
Man-made structures was changed the natural behaviour of the watershed. Such components like barrage, reservoir and diversions included to the system.
2.2 Methodology
The complex watershed model developed using Rainfall-Runoff and Inundation (RRI) Model (Sayama, 2013) by combining:
-
Observed data used for physical activities like, Rainfall, Topography, infiltration, and MODIS (or Moderate Resolution Imaging Spectroradiometer) satellite data for Evapotranspiration. For Non availability of rainfall data JAXA (Global Satellite Mapping of Precipitation) GSMaP data used after biased correcting.
-
Reservoir operations, based on monthly-based flood storage concept for different reservoirs, according to its climatic behaviour, like seasonal rainfall characteristics, to reduce floods in lower segment of the basin.
-
Four-stage of reservoir operations, such as pre-flood operation stage, flood operation stage, pre-emergency operation stage, and emergency operation stage. Spill operation combined with fuzzy logic technic with linear operation.
-
Monthly-based water diversion, which calculating the demand by using water requirement in the dry zone.
Two FORTRAN Modules, which is Dam Operation Module (DOM), that developed to include the monthly-based reservoir operation technic and four-stage reservoir operation technic, and Diversion Module (DM), that developed to include the monthly based water requirement in dry zone. Additionally CROPWAT 8.0, which developed by Food and Agriculture Organization (FAO), used to find out the irrigation water requirement of paddy and other field crops (Surendran, 2015). Finally, validated Mahaweli basin RRI model coupled with the two modules, that used for the complex watershed management as Complex System Approach Model (CSAModel).
2.3 Case Study: Mahaweli river basin in Sri Lanka
The developed CSAModel applied to Mahaweli river basin to verify the results and check its performance of the model, because Mahaweli basin complex due to:
-
River flows through different climatic zones.
-
Existing Reservoirs and water Diversions.
-
Inundation in lower part with huge property and agricultural damage.
-
Dry zone water scarcity.
-
Frequent confliction between the users and stakeholders.
The developed CSAModel applied to overcome the above complex behaviour in watershed.
2.3.1 CSAModel for Mahaweli river basin
Basic hydrological model prepared using RRI Model and 30Arcsec grid Digital Elevation Model (DEM) data. Model coupled with hydrological processes, spatial and temporal variation of rainfall distributions (Hydrological System), topographic change, sub-basin distribution and water contribution to mainstream (Geological System), water diversion locations, reservoir locations and flood situation (Man Made System). Considering above factors system divided to upper river basin (Eq. 1), Aban river basin (Eq. 2) and lower river basin (3) as shown in Fig. 1.
40 years (1975–2015) ground rainfall data used as temporal data in 15 stations, those data spatially interpolated by using Inverse Distance Weighting (IDW) method “Eq. (1)” this method gave the additional advantage to system for better results. Satellite based Evaporation data used after bias correcting with available ground data as in “Eq. (2)”
Where Zi Interpolation value in an unknown cell, dij distance between the known cell and unknown cell, Zj Known cell Value, P power function for weighting of the distance for this it takes as 6.
Where, Psat, satellite-observed data, μ(Psat) mean of the satellite data and, μ(Pob) mean of the observed data.
The developed basic Hydrological Model calibrated and validated for several flood events within 1969–1972 period, without considering reservoir and water diversion, because basin development started after 1972. During the model preparation stage it was used the three stage modelling, which considering Peradeniya, Mahiyanganaya and Mananpitiya Stations (Fig. 1) step by step from upper basin to lower basin. This was very effective for better performance of the model, because physical behaviours like river profile, multiple land use patterns, and soil parameters vary from the upper basin to lower basin. The Nash-Sutcliffe Efficiency (NSE) (Nash and Sutcliffe, 1970) value used for check the efficiency of Model.
2.3.2 CSAModel with Dam and Diversion
The developed basic Hydrological Model converted to CSAModel by combining Dam Operation Module (DOM) and Diversion Module (DM), which represent present condition of the Mahaweli watershed, this CSAModel further analysed and validated using existing operation sequence of the reservoir and diversion. This used to develop of new set of rules to achieve better results by considering following watershed characteristic and conditions.
-
The way of reservoir operation is key task for controlling flood in lower basin (Hoang, 2017) which can control the loss of property, economic and agricultural damage.
-
Analysed and identified the significance of spatial (Fig. 2) and temporal (Figs. 3 and 4) variation of the rainfall in watershed considering past 40 years data (1975–2015).
-
Identified the required flood storage capacity in the reservoir in monthly wise, by studying of annual spatial variation of rainfall (Fig. 2).
2.3.3 Flood Operation characteristic during monsoon
Lower basin scatted with dry zone reservoirs, which need water from wet zone, to fulfil the dry condition and upper basin consist with major reservoirs to control flood, water diversion and hydropower generation. The study identified that, spatial and temporal variation of the rainfall throughout the watershed, then it analysed, and categorized the reservoirs to two groups as follows.
Upper part get more rainfall during the June, July, August and September months (Fig. 4), due to South West Monsoon (SWM) (Fig. 2b). Therefore, Kothmale Reservoir selected as SWM flood reservoir and decided to maintain the flood storage as 15 %, 20 %, 20 % and 15 % from the gross storage, during these months. However, the lower part gets high rainfall from October, November and December months (Fig. 3), during North East Monsoon (NEM) (Fig. 2a), therefore lower part reservoirs were selected as NEM flood reservoirs, during the October, November, and December months and kept 20 % of flood storage in Victoria, Randenigala, Rantembe, and Bowathenna reservoirs.
The modified monthly-based flood storage used for reservoir operation, as mentioned above from January to December.
This flood storage divided to main four sections for control the reservoir, by considering the reservoir operation technics, then four-stage reservoir operation technic was introduced during the identified flood period as follows (see Fig. 5).
-
Pre-Flood Operation Stage, when reservoir water level fill to this level, reservoir operation will activate.
-
Flood Operation Stage, controlled spilling discharge to minimize the damage to lower basin.
-
Pre Emergency Operation Stage, indicate the to lower basin minor flood during the operation.
-
Emergency Operation Stage, the out flow will control for safety of the dam, with the operation rules.
This optimization technic introduced to the five major reservoirs in Mahaweli watershed, which Kothmale, Victoria, Randenigala, Rantembe, and Bowathenna as flood controlling reservoirs.
Diversion Module (DM) used for Trans-basin water diversion, which fulfil the dry zone water requirement. In our case study, actual diversion points in the stream introduced to module for dry zone diversion that started from Polgolla barrage to four major watersheds, which is outside of Mahaweli watershed, Kala Oya, Malwathu Oya, Yan Oya and Maduru Oya basin, altogether this diversion provide water to cultivate more than 165 000 ha agricultural lands in the dry zone. Other diversion locations are Minipe, Elahera and Mawilaru.
2.4 Data used
-
Rainfall data from 1965 to 2015 used
-
Ground Observed Discharge data (1965–2015) in Peradeniya, Mahiyanganaya and Mananpitiya station (see Fig. 1).
-
MODIS satellite evapotranspiration data (2014–2015), and ground observed evaporation data (2014–2015) in Palugasdamana and Mahailippallama (Hydrological Annual, 2009–2015).
-
Observed Reservoir operation data (2014–2015) in Bowathenna, Victoria, Randenigala, Rantembe, and Kothmale.
Basic hydrological model was calibrated (NSE value 0.61) and validated (NSE value 0.76) by using data 1969–1972, which is no dam and diversion in the watershed. Pre-optimized CSAModel simulated and check the results, one by one in each reservoirs with actual operation data with the real flood situation in lower basin for validation the CSAModel.
3.1 Optimized dam operation for flood
Monthly based flood storage and four-stage dam operational rules applied to CSAModel and reservoir water discharge and storage change observed with the lower part flood in 2014 event. During the simulation flood storage, diversion capacity was adjusted for minimum inundation of the lower basin and keep the maximum storage after the flood events.
Figure 6a and b curves shows that observed discharge and the modelled discharge with original operation in lower part. Figure 6c shows that performance of newly proposed four-stage dam operational rules. When comparing the both results, it shows that flood peak in Mananpitiya area reduced from 2800 to 1450 m3 s−1 according to newly introduced flood storage system. That reduced the actual flood level by 1.5 m in lower basin. Figure 7 shows the reduction of the inundation area. Figure 8 indicate that how new flood storage applied and varied during the months, and reservoir capacity variation according to new CSAModel in Victoria reservoir. This was applied for all five reservoirs, which I mentioned earlier.
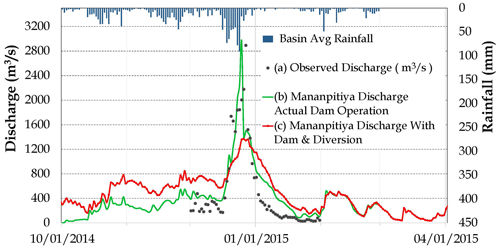
Figure 6Discharge change (a). Actual Dam Operation (b). Observed data (c). Application of new set of rules for dam operation.
3.2 Optimized diversion for drought
The developed CSAModel diversion function used to optimize the water diversion in dry zone. In Mahaweli watershed, trans-basin diversion (Fig. 9) stared from Polgolla barrage to dry zone through tunnel. Water diversion is not enough for dry zone. Therefore, during the study two different scenario compared to optimize the maximum diversion, by changing tunnel capacity (S01) and using same tunnel (S02). Model output shown that 21 % of cultivation lands could have increased in 2014 Yala, and 17 % in 2015 Yala, for S01, and. 10 % of cultivation lands could have increased in 2014 Yala and 6 % in 2015 Yala for S02 in dry zone. This diversion optimization shows that rice production increment in dry zone, if used such optimization, and it will save USD 15–20 million per year.
Watersheds are more complex to predict, even it more complex due to man made changes in the system, during the COVID period it was more difficult due to unavailability of proper system. During the several decades, different studies carried out to find the watershed management tool, which can used for forecast and reduce the disasters in watershed as discussed earlier. In this study, CSAModel developed to address this by using following steps, (a) identifying of Natural processes such as hydrological processes, topography, spatial and temporal variation of rainfall distributions and evapotranspiration, (b) simplification of complex watershed, (c) introducing physical processes and water storage and diversion facilities and, (d) application of the combined complex hydrological model.
The CSAModel applied to complex Mahaweli watershed, which is largest in Sri Lanka, to check the efficiency of the system and take the output for reduce the disasters, efficiency management, and decision making for stakeholders. The developed CSAModel performed well by selecting the spatially varied flood storage and four-stage dam operation rules. Finally model output shows that considerable amount of flood reduction in lower basin. The capacity of flood storage reduction after the event is very effective to increase the water diversion to dry zone and it shows that increase of paddy cultivation in Yala season.
Further, the study identified that, flood reservoirs for flood management, and some reservoirs for drought management according spatial variation and temporal variation of the rainfall in watershed. The model flexible for use the optimum hydropower generation with maximum cultivation performance after some modification. Finally, CSAModel helps for integrated water resource management (IWRM) in watershed by sharing data among the stakeholders for decision-making.
The study recommend that to form new committee among the shareholders e.g., Hydropower, Irrigation and drinking sector. Additionally, coupling of seasonal prediction models and checking of water availability in the system is added advantage of this model. Therefore, study recommend for coupling CSAModel with seasonal rainfall forecasting model for better forecast and manage the whole system as one unit with stakeholders during this type of Pandemic situation.
Code developed based on the Open Source software called RRI Modeling developed by ICHARM (https://www.pwri.go.jp/icharm/research/rri/rri_top.html, Sayama, 2013), modified the existing version of the code to include the new Dam Operation Module (DOM) and Diversion Module (DM) to it.
The hydrological data, including river flow, rainfall, and evaporation records, is officially owned by the Department of Irrigation and the Meteorology Department. This data, covering the period from 1965 to 2015 within the Mahaweli Watershed, is designated for research purposes and can be accessed through a formal written request to the respective departments.
The authors confirm contribution to the paper as follows: study conception, data collection, analysis, interpretation of results and draft manuscript preparation: RIJ. MR, TK: reviewed the results and approved the final version of the manuscript.
At least one of the (co-)authors is a guest member of the editorial board of Proceedings of IAHS for the special issue “ICFM9 – River Basin Disaster Resilience and Sustainability by All”. The peer-review process was guided by an independent editor, and the authors also have no other competing interests to declare.
Publisher’s note: Copernicus Publications remains neutral with regard to jurisdictional claims made in the text, published maps, institutional affiliations, or any other geographical representation in this paper. While Copernicus Publications makes every effort to include appropriate place names, the final responsibility lies with the authors.
This article is part of the special issue “ICFM9 – River Basin Disaster Resilience and Sustainability by All”. It is a result of The 9th International Conference on Flood Management, Tsukuba, Japan, 18–22 February 2023.
The authors would like to thank the constructive comments from reviewers, and special thanks to coauthors, Mohamad Rasmy (Senior Researcher, ICHARM), and Toshio Koike (Director of ICHARM/Professor Emeritus of the University of Tokyo) for guiding to research. Further, I would like to express my gratitude to Director General of Irrigation and his staff for supporting the research by allowing me to use relevant data. Also, I am deeply grateful to the Japan International Cooperation Agency (JICA), Graduate Institute of Policy Studies (GRIPS), Public Work Research Institute (PWRI) and International Center for Water Hazard and Risk Management (ICHARM) for their support. Finally, I wish to extend my gratitude to my parent, loving wife and all others for their noble supports.
This paper was edited by Kensuke Naito and reviewed by two anonymous referees.
Hoang, N. V.: Analysis of Effective Reservoir Operation for Mitigating Flood Damages in a Vuong Dam Basin, ICHARM Master Paper No. 119, https://www.pwri.go.jp/icharm/training/master/img/2017/synopses/mee16728.pdf (last access: 6 July 2024), 2017.
Hydrological Annual: Irrigation Department of Sri Lanka, Irrigation Department, https://www.irrigation.gov.lk/web/index.php?option=com_content&view=article&id=115&lang=en (last access: 6 July 2024), 2009–2015.
Khan, S., Yufeng, L., and Ahmad, A.: Analysing complex behaviour of hydrological systems through a system dynamics approach, Environ. Modell. Softw., 24, 1363–1372, https://doi.org/10.1016/j.envsoft.2007.06.006, 2007.
Nash, J. E. and Sutcliffe, J. V.: River Flow Forecasting through Conceptual Models Part I – A Discussion of Principles, J. Hydrol., 10, 282–290, https://doi.org/10.1016/0022-1694(70)90255-6, 1970.
Sajjad, A. and Slobodan, P. S.: System Dynamics Modelling Of Reservoir Operations For Flood Management, J. Comput. Civil Eng., 14, 190–198, https://doi.org/10.1061/(ASCE)0887-3801(2000)14:3(190), 2000.
Sayama, T.: Rainfall-runoff-inundation (RRI) model ver 1.4.1, International Center for Water Hazard and Risk Management (ICHARM) and Public Works Research Institute (PWRI) [code], https://www.pwri.go.jp/icharm/research/rri/rri_top.html (last access: 6 July 2024), 2013.
Surendran, U.: Modelling the crop water requirement using FAO-CROPWAT and assessment of water resources for sustainable water resource management: A case study in Palakkad district of humid tropical Kerala, International Conference on Water Resources, Costal and Ocean Engineering (ICWRCOE 2015), Mangalore, India, https://doi.org/10.1016/j.aqpro.2015.02.154, 2015.
Xin, L.: Watershed system model: The essentials to model complex human-nature system at the river basin scale, J. Geophys. Res.-Atmos., 123, 3019–3034, 2018.