the Creative Commons Attribution 4.0 License.
the Creative Commons Attribution 4.0 License.
Impact of the operation of cascade reservoirs in upper Yangtze River on hydrological variability of the mainstream
Xu Changjiang
Zhang Dongdong
As the impacts by climate changes and human activities are intensified, variability may occur in river's annual runoff as well as flood and low water characteristics. In order to understand the characteristics of variability in hydrological series, diagnosis and identification must be conducted specific to the variability of hydrological series, i.e., whether there was variability and where the variability began to occur. In this paper, the mainstream of Yangtze River was taken as the object of study. A model was established to simulate the impounding and operation of upstream cascade reservoirs so as to obtain the runoff of downstream hydrological control stations after the regulation by upstream reservoirs in different level years. The Range of Variability Approach was utilized to analyze the impact of the operation of upstream reservoirs on the variability of downstream. The results indicated that the overall hydrologic alterations of Yichang hydrological station in 2010 level year, 2015 level year and the forward level year were 68.4, 72.5 and 74.3 % respectively, belonging to high alteration in all three level years. The runoff series of mainstream hydrological stations presented variability in different degrees, where the runoff series of the four hydrological stations including Xiangjiaba, Gaochang and Wulong belonged to high alteration in the three level years; and the runoff series of Beibei hydrological station in 2010 level year belonged to medium alteration, and high alteration in 2015 level year and the forward level year. The study on the impact of the operation of cascade reservoirs in Upper Yangtze River on hydrological variability of the mainstream had important practical significance on the sustainable utilization of water resources, disaster prevention and mitigation, safe and efficient operation and management of water conservancy projects and stable development of the economic society.
- Article
(2577 KB) - Full-text XML
- BibTeX
- EndNote
Under the impacts by climate changes and human activities, the characteristics of meteorological and hydrological elements in many regions of the world had presented changes in different degrees (Guo et al., 2012). The globally observed evaporation pan evaporation was basically on the decline while the actual evaporation presented in an increasing trend (Kisi, 2015; McVicar et al., 2012). The precipitation changing trend displayed strong regional characteristics (Allan, 2012; Prakash et al., 2015; Wen et al., 2015). Sun and Ao (2013) pointed out that the precipitation changing trend in China in recent 100 years was not obvious; but a slight increasing trend appeared since 1956; and in the future 20–100 years, the annual precipitation in China would appear in an increasing trend. Based on the data observed in about 730 meteorological stations during 1951–2002, the precipitation changes in China displayed strong regional characteristics, displaying a continuous declination in northern China, central China and southern regions in northeastern China and obvious increase in the regions to the south of the Yangtze River basin (Jiang et al., 2014). The studies by Barnett et al. (2008) showed that the water circulation in the western United States had changed dramatically in the second half of the 20th century, where as high as 60 % was due to the environmental changes caused by human activities. The study by Szilagyi (2001) discovered that the mean runoff depth of Republican River in the US during 1977–1996 decreased by around 40 % compared to that in 1949–1968; and this decrease was mainly the result of affections by comprehensive human activities in the basin like reservoir construction, agricultural irrigation, vegetation change and soil and water conservation, etc. The studies by many Chinese scholars showed that the runoff in northern regions of China appeared in a decreasing trend in recent 50 years; for example, the reason of continuous decrease in the actual inflow water amount of the mainstream of Yellow River in recent 50 years was mainly due to the impacts by climate changes and human activities (Liu and Cheng, 2000). The long-series data observed in basins of Yellow River, Hai River, Liao River and Songhua River showed that the river runoff had a tendency to decrease and the runoff during the 1980s and 1990s had decreased by 20.50 % than that during the 1950s and 1960s under the same magnitude of rainfall (Ren et al., 2001). It was found after analyzing the annual runoff series of the Yellow River of nearly 80 years with the detrended fluctuation analysis method that there was an inherent long-range correlation in the annual runoff of the Yellow River of nearly 80 years; and the trend prediction showed that the runoff change of the Yellow River in next ten years and more may appear in a decreasing trend (Li et al., 2008). The data of some basins in southern China regions in recent 50 years showed that the underlying surface changes had resulted in the runoff increase; for example, comparing the runoff in the 1980s and 1990s to that in 1970s, the runoff of the Bei River basin of the Pearl River system increased by 7–10 % (Li et al., 2006) and the runoff of Dong River basin increased by 25.7 % (Lin et al., 2012).
The river ecosystem plays an extremely important role in maintaining regional water circulation, energy balance, climate change and ecological development and is also the most important human life support system, providing water for production, living and ecology. In the 1980s, the “river health management” was on the rise in Europe and North America; and in 2002, the Chinese experts and scholars put forward the idea of “a healthy river ecosystem is bound to become the main target of river management” while introducing the concept of “river health” (Petts, 1979, 1980). The researchers from all over the world had conducted a lot of studies on water requirement of river ecology and environment since the 1970s and had produced many calculation and evaluation methods (Pettit et al., 2001; Poff et al., 1997). In 2003, Tharme (2013) conducted some statistics and found out 207 kinds of ecological flow requirement assessment methods in 44 countries, which could be roughly divided into four categories: hydrologic indicator method (historical flow method), hydraulic method, habitat method (ecological environment) and holistic method.
The river ecosystem is a dynamic flowing water system possessing double attributes in nature and society respectively. Its integrity is largely dependent on the natural dynamic change characteristics of water flow, namely, the river's hydrological regime. The construction of hydraulic structures like reservoir and dam was mainly for the purpose of flood control, navigation and water supply, which also impacted the hydrological regime of downstream river channel (Mathlouthi and Lebdi, 2008). Richter et al. (1996) established a set of indicator system (Indicators of Hydrologic Alteration, IHA) to assess the changing conditions of river hydrology before and after the interference by human activities through a total of 32 indicators in five aspects namely flow, occurrence time, frequency, duration and change rate, and proposed the RVA method (Range of Variability Approach) based on IHA (Richter et al., 1997) for setting the flow of ecological environment as well as the goals and steps of river management, having laid a foundation for analyzing the river's hydrological regime. Richter et al. (1998) applied the RVA method in assessing the hydrological variability before and after the dam construction on the main rivers in the Colorado River basin. The RVA method was regarded as an effective method to assess river's hydrological variability (Chen et al., 2010). Shiau and Wu (2004) used RVA method to investigate the hydrologic conditions before and after the construction of a diversion weir on Chou-Shui Creek, Taiwan, suggesting that restoration of the natural flow is expected to promote the natural stream biota. The RVA has proved to be a practical and effective approach facilitating river restoration planning. However, the RVA method is only used to assess the small-basin rivers in assessing the river's hydrological regime changes, lacking of assessment on the overall alteration conditions of large-basin rivers. To this end, this paper adopted the RVA method, incorporated the runoff regulation and calculation model, and selected the main control hydrological station on the mainstream of Yangtze River to study the impact of the operation of cascade reservoirs in Upper Yangtze River on hydrological variability of the mainstream.
Being rich with hydraulic power potentials, dense river network, abundant rainfall and large runoff volume, the upper Yangtze River basin is the most important rich water area. According to the statistics from “Electric power planning in China (2002)”, 34 large reservoirs had been built up or under construction on the upper Yangtze River, and more than 30 large reservoirs had been designed and set up with a total storage capacity of about 200 billion m3. Since 2000, large reservoirs were constructed mainly in the lower reaches of the Jinsha, Yalong, Dadu and Wujiang Rive basin. In 2020, the layout of major rivers cascade reservoirs will be basically completed in the Yangtze River. Even before, the hydrological cycle was based on precipitation, surface runoff, river and groundwater. But now, the cycle most is also impacted by the strong human activities in four aspects: water taking, water transporting, water usage, drainage and regress (Jia et al., 2006).
To a certain degree, the impacts of human activities on hydrological processes are even more serious than the impacts of climatic change in the upper Yangtze River. Exploring the extent to which human interventions affected the hydrological regimes and related hydrological alterations is crucial for better understanding of human-induced hydrological alterations, and will aid water resources management within the Yangtze River basin. The objectives of this paper therefore are: (1) to present a visually enhanced hydro-alteration assessment method for flow of Yangtze River with data series encompassing pre- and post-alteration periods; (2) to determine the spatial behavior of 33 Indicators of Hydrologic Alteration (IHA) factors which features the hydrologic alteration in Yangtze River; and (3) to evaluate the impact of dams on the hydrological alteration along the Yangtze River of China.
There are numerous reservoirs on the mainstream and tributaries at the upstream of the Three Gorges. Based on the runoff characteristics of upper Yangtze River, the reservoirs with high regulation capability were selected as the objects of this study. According to the statistics of water resources bulletins of the Yangtze River basin and rivers in the southwest, the construction and impoundment of large and medium-sized reservoirs upstream the Three Gorges had been completed successively during 2003–2013, with total accumulated impoundage of 8.66 billion m3. The upstream large and medium-sized reservoirs are mainly for the purpose of annual regulation or seasonal regulation, and the impoundment quantity is mainly the dead reservoir capacity. The impoundment of Three Gorges Reservoir starts from 15 September to the end of November. The total impoundment quantity needed for the constructed large reservoirs in the basin upstream the Three Gorges is 10.33 billion m3; 5.11 billion m3 for the reservoirs under construction; and 7.08 billion m3 for the proposed reservoirs; 22.52 billion m3 in total. As for the selection of upstream reservoirs in this study, in aspect of capacity, the large reservoirs with a single reservoir regulation capacity of more than 500 million m3 were selected as the objects of study; in aspect of operating time, the reservoirs constructed and put into operation before 2015 were mainly considered. As for the reservoirs constructed after 2015, because of the many variable factors, only the recently developed reservoirs recommended in the comprehensive planning of Yangtze River basin were considered.
According to the aforementioned principles, the reservoirs of Lianghekou, Jinping First-cascade and Ertan on Yalong River, Wudongde, Baihetan, Xiluodu and Xiangjiaba on Jinsha River, Zipingpu on Min River, Pubugou and Shuangjiangkou on Dadu River, Baozhusi on Bailong River, Tingzikou on Jialing River and Hongjiadu, Wujiangdu, Goupitan and Pengshui on Wu River were selected as the objects of study (Fig. 1 and Table 1).
At present, the upstream reservoirs have not been completely constructed; only the constructed reservoirs are under operation at the upstream in current stage; at the same time, the reservoirs under construction will be constructed and put into operation in succession where most of the reservoirs under construction will be constructed around 2015; and the construction completion and production time of the reservoirs planned to be constructed will be even later. Therefore, the constructed reservoirs, reservoirs under construction and proposed reservoirs shall be differentiated appropriately according to their time put into production. In this study, in order to differentiate the production time of the constructed reservoirs, reservoirs under construction and proposed reservoirs, it was divided into three level years according to the reservoir's construction completion status, namely, 2010 level year, 2015 level year and the forward level year. The reservoir construction completion status considered in each level year is as follows.
3.1 Indicators of hydrologic alteration
The Indicators of Hydrologic Alteration (IHA) approach is a method to assess river's ecological hydrologic alterations. Mainly based on such 5 basic characteristics of hydrological regime as flow, occurrence time, frequency, duration and change rate, the IHA approach is divided into 33 indicators in five groups according to the characteristics summarized (as shown in Table 2). The relationship between the parameters of each IHA group and the river ecosystem is as shown in the literature (Richter et al., 1997).
The RVA approach is to determine the river flow status before and after alteration by utilizing the detailed flow data on the basis of IHA, so as to analyze the alteration before and after the river alteration (Richter et al., 1997). The assessment can be conducted in the following steps:
-
Calculate the characteristic values of 33 IHAs by utilizing the daily flow data before alteration;
-
Define the target RVA range of each IHA according to the calculation results of previous step; and the values of the occurrence probabilities of each IHA before alteration of 75 and 25 % were taken as the target RVA range in this paper;
-
Calculate the characteristic values of 33 IHAs by utilizing the daily flow data after alteration;
-
Use the RVA threshold values obtained in Step (2) to judge the alteration of the river's hydrological regime after alteration, determine its impact and represent it with the overall hydrologic alteration. The hydrologic alteration is defined as follows:
Where: Di is the hydrologic alteration of number i IHA; Y0i is the number of years of number i IHA in the range of RVA after alteration; Yf is the number of years of IHA in the range of RVA after alteration. Generally, the value of Di is divided into three sections: 0–33.3 %, low alteration; 33.3–66.7 %, medium alteration; 66.7–100 %, high alteration.
The response degrees of the aforementioned 33 IHAs before and after alteration are not the same and it needs to conduct an overall assessment on the alteration of river's hydrological regime. The overall hydrological characteristic variability is represented with the overall hydrologic alteration D0 and the actual assessment method is as follows:
It shall be categorized as low alteration when all 33 IHAs belong to low alteration:
It shall be categorized as medium alteration when at least one IHA belongs to medium alteration but no IHA belongs to high alteration:
Where: Nm is the number of IHA belonging to medium alteration.
It shall be categorized as high alteration when at least one IHA belongs to high alteration:
Where: Nh is the number of IHA belonging high alteration.
3.2 Runoff regulation and calculation model
Through establishing a model to simulate the impounding and operation of upstream cascade reservoirs, the runoff of downstream hydrological control stations after the regulation by upstream reservoirs is obtained in different level years, and further the impact of the operation of upstream reservoirs on downstream hydrological variability is analyzed. The runoff regulation is calculated with the “Combined Reservoir Group Water Regulation Model Software 1.0”; the main model structure (Fig. 2) are as follows.
First step, the model first restores the actually measured runoff series affected by the reservoir regulation and impoundment and also obtains the natural runoff series of each hydrological control station and reservoir dam site through the restoration;
Second step, the reservoir at the highest reach of each branch of the upper reach conducts regulation on the natural runoff at dam site, and conducts routine regulation and operation according to the regulation rules, thus obtaining the runoff series after operation; the runoff series after operation is calculated downwards level by level to the reservoirs of the lower levels, and then regulated and operated by the lower-level reservoirs thus obtaining the runoff series of the hydrological control station of each branch after regulation by upstream reservoirs ultimately through the operation level by level.
The model mainly consists of the runoff restoration, upstream reservoir regulation and operation, and runoff calculation modules. The model structure is as shown in Fig. 3.
3.2.1 Runoff restoration module
Since there are some large reservoirs already constructed at the upstream of the Three Gorges and the operation of these reservoirs has changed the natural situations of the runoff of river channels concerned, in order to achieve consistency in the analyzed and calculated runoff series, the runoff needs to be restored.
The restoration calculation of this module is mainly to calculate reservoir's daily (or pentad) storage variables with the reservoir water balance equation and according to the reservoir's dam water level as well as inflow and outflow. The details are as shown in Eq. (1):
Where: Qinflow is the mean reservoir inflow in the time period; Qoutflow is the mean reservoir outflow in the time period; Qled is the mean reservoir led-in or led-out flow in the time period; ΔW is the reservoir water storage change value in Δt time period; ΔWloss is the reservoir water loss quantity (including the evaporation and seepage quantity) in Δt time period; Δt is the length of time period.
To simplify the calculation, the reservoir water loss quantity ΔWloss was not considered this time, therefore:
Where: Zt and Zt+1 is the reservoir water level at the beginning and end of t time period respectively; V(Zt) and V(Zt+1) is the reservoir capacity at the beginning and end of t time period respectively.
Take as the mean reservoir water storage flow, then:
In the case of the reservoir diversion water flow not considered, ΔQ represents the reservoir impoundment when it is positive, and represents that the reservoir is utilizing the regulated reservoir storage capacity to increase the discharge when it is negative.
3.2.2 Upstream reservoir regulation and operation module
The upper Three Gorges reservoir regulation and operation module is to simulate the normal regulation and operation of upstream reservoirs, simulate the reservoir operation according to the operation chart and dam-site runoff of each reservoir, and calculate the reservoir water storage variation in each time period and the alterations of natural runoff after the operation of reservoir. During the reservoir regulation and operation simulation, the operation was conducted in strict accordance with the reservoir operation chart based on the reservoir water level at the beginning of the time period and the reservoir inflow water within the time period. The simulation time period was very long; therefore, the reservoir flood control regulation was not considered in the module. At the same time, in this study, all reservoir operations were based on the regular operation of the operation chart, and the emergency regulation of each reservoir under special circumstances was not considered.
3.2.3 Runoff calculation module
Either in the case of restoring the water storage variable of each reservoir in the calculation or simulating the upstream reservoir runoff after regulation, the calculation needs to be conducted downstream to the reservoirs or hydrological control stations of the lower levels so as to obtain the runoff of downstream hydrological control station or reservoir after the restoration and that after the regulation by upstream reservoirs. The runoff calculation module is mainly used to simulate the calculation of the runoff of upstream reservoirs or hydrological control stations to the downstream. The calculation time period of this study was very long and belonged to long-series operation, having not only a very long time span, but also the flood season and the dry season. Currently, the studies on the dry season runoff calculation are relative few and are not mature in terms of methodology compared to the flood season flood evolution. This time, it was disposed with a simplified method where only the propagation time between two hydrological stations was considered, then simple transposition was conducted on the runoff process without taking into consideration the attenuation and deformation, and the upstream flow after transposition plus interval flow was taken as the downstream flow. The propagation time determination was mainly based on the flow and flow velocity in September–October of the water storage period. Since the time period for the reservoir regulation simulation in this time was a long-wait period, the propagation time analyzed was also relatively rough, taking the day as the unit. Upon analysis, the propagation time from upstream reservoirs to the hydrological control station of each branch and Yichang considered in this time of calculation was as follows:
-
Min River: the propagation time was 2d (days) from Pubugou Reservoir to Gaochang, 2d from Zipingpu Reservoir to Gaochang also, and 3d from Gaochang to Three Gorges Reservoir.
-
Jinsha River: the propagation time was 1d from Ertan Reservoir to Wudongde Reservoir, 2d from Wudongde Reservoir to Xiluodu Reservoir, 1d from Xiluodu Reservoir to Xiangjiaba Reservoir and 3d from Xiangjiaba Reservoir to Three Gorges Reservoir.
-
Jialing River: the propagation time was 1d from Baozhusi Reservoir to Tingzikou Reservoir, 3d from Tingzikou to Beibei; and 1d from Beibei hydrological station to Three Gorges Reservoir.
-
Wu River: the propagation time was 1d from Hongjiadu Reservoir to Wujiangdu Reservoir, 1d from Wujiangdu Reservoir to Goupitan Reservoir, 1d from Goupitan Reservoir to Pengshui, and 1d from Wulong to Three Gorges Reservoir. The propagation time from Pengshui to Wulong was not considered due to its shortness.
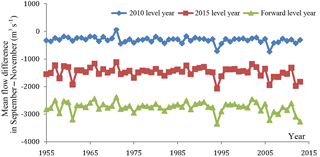
Figure 4Process of mean flow of Yichang hydrological station from September to November in different level years and the natural condition.
3.2.4 Model calculated series and time period
Since there are many reservoirs on the upper Yangtze River, the design of each hydrological control station and reservoir on each branch is inconsistent due to the different length of series observed in each station, with the shortest starting from 1955 and the observation data missing in some years. The synchronized series of each hydrological station in 1955–2013 was simulated and calculated, and the series of several hydrological stations in the years absent of observation was supplemented with the upstream and downstream runoff or the station's water level. Therefore, all runoff series after the operation of reservoirs mentioned in this study refers to the series in 1955–2013.
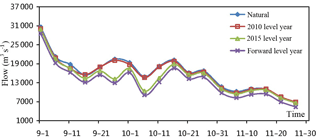
Figure 7Process of Yichang hydrological station from September to November in slightly dry year (1976).
In the runoff impact simulation, the calculated time period was controlled by five days. During the operation simulation of each reservoir, the corresponding regulation and operation was all conducted according to the operation chart based on the initial reservoir water level of every five days and the inflow water at the moment.
4.1 Hydrological variability analysis of Yichang hydrological station
In order to analyze the impact of large reservoirs at the upstream of Three Gorges on the hydrological variability of Three Gorges Reservoir inflow runoff, the runoff of Yichang hydrological station was taken in representation of the Three Gorges Reservoir inflow runoff. The regulation and impoundment functions of Three Gorges Reservoir were not considered in the three level years.
The mean flow of Yichang hydrological station form September to November in different level years is as shown in Table 3.
The mean flow from September to November after the operation of reservoirs decreased to different degrees; the mean flow from September to November in 2010 level year was 16 900 m3 s−1, decreased by 300 m3 s−1 compared to the natural condition of 17 200 m3 s−1 and achieved a decreasing amplitude of 1.8 %; the mean flow from September to November in 2015 level year was 15 800 m3 s−1, decreased by 1400 m3 s−1 compared to the natural condition and achieved a decreasing amplitude of 8.6 %; the mean flow from September to November in the forward level year was 14 500 m3 s−1, decreased by 2700 m3 s−1 compared to the natural condition and achieved a decreasing amplitude of 16 %.
Figures 4–8 are the comparison of the pentad flow of Yichang hydrological station representing different situations. The operation of reservoirs had a relatively small impact on the runoff in 2010 level year; however, in 2015 level year and the forward level year, the impact increased gradually as the reservoirs of Xiluodu, Xiangjiaba, Jinping First-cascade, Goupitan, Pengshui, Wudongde and Baihetan were put into operation in succession; in the forward level year, the mean flow in September–November after the operation of reservoirs in the slightly rainy year was smaller than that of the natural condition by 14.4 % or so and that in the especially dry year amounted to 28.6 %.
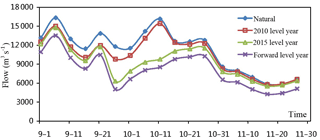
Figure 8Process of Yichang hydrological station from September to November in especially dry year (2006).
The RVA calculation results of hydrological variability of Yichang hydrological station in different level years are as shown in Table 4. It can be seen that the overall hydrologic alterations in 2010 level year, 2015 level year and the forward level year were 68.4 72.5 and 74.3 % respectively, all belonging to high alteration in the three level years.
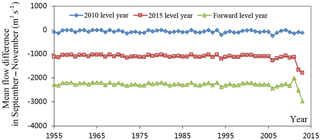
Figure 9Process of mean flow of Xiangjiaba Hydrological Station from September to November in different level years and the natural condition.
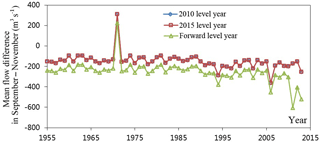
Figure 10Process of mean flow of Gaochang hydrological station from September to November in different level years and the natural condition.
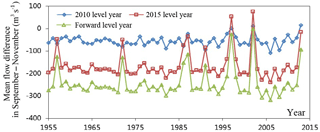
Figure 11Process of mean flow of Beibei hydrological station from September to November in different level years and the natural condition.
4.2 Hydrological variability of other hydrological stations
The difference process of the mean flow of hydrological control stations on the mainstreams of Yangtze River from September to November in different level years and the natural condition is as shown in Figs. 9–12. The RVA calculation results of hydrological variability of the hydrological stations on each mainstream in different level years are shown in Table 5.
Table 5RVA calculation results of hydrological variability of mainstream hydrological stations in different level years.
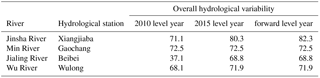
The operation conditions of the reservoirs on Jinsha River in different level years are as follows: only Ertan Reservoir was operated in 2010 level year; the three reservoirs of Xiluodu, Xiangjiaba and Jinping First-cascade will be newly put into operation in 2015 level year; and the Lianghekou, Wudongde and Baihetan Reservoirs will be put into operation in the forward level year. The overall hydrologic alterations in 2010 level year, 2015 level year and the forward level year were 71.1, 80.3 and 82.3 % respectively, all belonging to high alteration.
Gaochang hydrological station is the outlet hydrological control station on Min River. Currently, there are two large reservoirs on Min River, namely, Pubugo Reservoir and Zipingpu Reservoir. In addition, Shuangjiangkou Reservoir on Dadu River is in the planning stage. Therefore, the two reservoirs of Pubugou and Zipingpu were operated at the same time in both 2010 level year and 2015 level year and Shuangjiangkou Reservoir will be newly put into operation in the forward level year. The overall hydrologic alterations in 2010 level year, 2015 level year and the forward level year were 72.5 % for all, all belonging to high alteration.
The outlet hydrological control station of Jialing River is Beibei hydrological station. Only Baozhusi Reservoir was operated on Jialing River in 2010 level year; Tingzikou Reservoir will be put into operation in 2015 level year; and Shuangjiangkou Reservoir will be put into operation in the forward level year. The overall hydrologic alterations in 2010 level year, 2015 level year and the forward level year were 37.1, 68.8 and 68.8 % respectively, belonging to medium alteration in 2010 level year and high alteration in 2015 level year and the forward level year.
Wulong hydrological station is the outlet hydrological control station of Wu River. The upstream reservoirs considered in this calculation are the four reservoirs of Hongjiadu, Wujiangdu, Goupitan and Pengshui. Only Hongjiadu and Wujiangdu Reservoirs were operated in 2010 level year; and the four reservoirs will be operated at the same time in both 2015 level year and the forward level year. The overall hydrologic alterations in 2010 level year, 2015 level year and the forward level year were 68.1, 71.9 and 71.9 % respectively, all belonging to high alteration in the three level years.
This paper mainly analyzed the reservoir impoundment quantity and evaporation loss and gain quantity of the constructed reservoirs and adopted the IHA approach to study the impact of the operation of cascade reservoirs in Upper Yangtze River on hydrological variability of the downstream. The main conclusions obtained are as follows:
For Yichang hydrological station, the mean flow from September to November after the operation of reservoirs decreased; the mean flow from September to November in 2010 level year was 16 900 m3 s−1, decreased by 300 m3 s−1 compared to the natural condition of 17 200 m3 s−1 and achieved a decreasing amplitude of 1.8 %; the mean flow from September to November in 2015 level year was 15 800 m3 s−1, decreased by 1400 m3 s−1 compared to the natural condition and achieved a decreasing amplitude of 8.6 %; the mean flow from September to November in the forward level year was 14 500 m3 s−1, decreased by 2700 m3 s−1 compared to the natural condition and achieved a decreasing amplitude of 16 %. The overall hydrologic alterations of Yichang hydrological station in 2010 level year, 2015 level year and the forward level year were 68.4, 72.5 and 74.3 % respectively, all belonging to high alteration in the three level years (The impact by the regulation and impoundment of Three Gorges Reservoir was not considered in all three level years). Similar results can be found in Zhang et al. (2012) which showed that reservoir operation in the upper Yangtze River decreases inflow to Three Gorges Reservoir at the end of flood season and increases that in dry season.
Under the impact by the operation of reservoirs and compared to the natural condition, the mean flow of Xiangjiaba hydrological station on Jinsha River from September to November decreased by 0.9 % in 2010 level year, 16.9 % in 2015 level year and 34.8 % in the forward level year; the mean flow of Gaochang hydrological station on Min River from September to November decreased by 4.9 % in both 2010 level year and 2015 level year and 7.9 % in the forward level year; the mean flow of Beibei hydrological station on Jialing River from September to November decreased by 2 % in 2010 level year, 5.9 % in 2015 level year and 8.9 % in the forward level year; the mean flow of Wulong hydrological station on Wu River from September to November decreased by 1.3 % in 2010 level year and 1.6 % in both 2015 level year and the forward level year; and the mean flow of Three Gorges Reservoir from September to November decreased by 1.8 % in 2010 level year, 8.6 % in 2015 level year and 16 % in the forward level year. The overall hydrologic alterations of Xiangjiaba hydrological station on Jinsha River in 2010 level year, 2015 level year and the forward level year were 71.1, 80.3 and 82.3 % respectively, all belonging to high alteration; the overall hydrologic alterations of Gaochang hydrological station on Min River in 2010 level year, 2015 level year and the forward level year were 72.5 % for all, all belonging to high alteration; the overall hydrologic alterations of Beibei hydrological station on Jialing River in 2010 level year, 2015 level year and the forward level year were 37.1, 68.8 and 68.8 % respectively, belonging to medium alteration in 2010 level year and high alteration in 2015 level year and the forward level year; and the overall hydrologic alterations of Wulong hydrological station on Wu River in 2010 level year, 2015 level year and the forward level year were 68.1, 71.9 and 71.9 % respectively, all belonging to high alteration in the three level years.
The construction and operation of reservoirs in the upper Yangtze River aims to reduce the flood disaster and sediment deposition but alters the downstream hydrological regime inevitably. In this paper, the RVA approach was used to assess the hydrological variability resulted before and after the dam construction in the upper Yangtze River. The results of this study will be greatly helpful for the future management of water resources and will be greatly important for further understanding of human impacts (e.g., hydro-dam projects) on hydrological regimes in the Yangtze River. Furthermore, it is significant for sustainable social and economic development in the Yangtze River which is one of the economically developed regions in China.
The research data is supplied by Bureau of Hydrology, Changjiang Water Resources Commission which can be obtain by visiting http://www.cjh.com.cn/.
The authors declare that they have no conflict of interest.
This article is part of the special issue “Innovative water resources management – understanding and balancing interactions between humankind and nature”. It is a result of the 8th International Water Resources Management Conference of ICWRS, Beijing, China, 13–15 June 2018.
This study is funded by the National Key Research and Development
Program of China (grant no. 2017YFC0405302-02).
Many thanks are given to the anonymous reviewers for their valuable comments.
Edited by: Dingzhi Peng
Reviewed by: two anonymous referees
Allan, R.: Regime dependent changes in global precipitation, Clim. Dynam., 39, 827–840, 2012.
Barnett, T. P., Pierce, D. W., Hidalgo, H. G., Bonfils, C., Santer, B. D., Das, T., Bala, G., Wood, A. W., Nozawa, T., Mirin, A. A., and Cayan, D. R.: Human-Induced Changes in the Hydrology of the Western United States, Science, 5866, 1080–1083, 2008.
Chen, Y. D., Yang, T., Xu, C., Zhang, Q., Chen, X., and Hao, Z.: Hydrologic alteration along the Middle and Upper East River (Dongjiang) basin, South China: a visually enhanced mining on the results of RVA method, Stoch. Environ. Res. Risk Assess., 24, 9–18, 2010.
Guo, L., Xia, Z., Yu, L., Wang, J., and Chen, Q.: Hydrological Variation Characteristics of Rivers in Humid Region: Oujiang River, China, Proc. Eng., 28, 95–98, 2012.
Jia, Y. W., Wang, H., and Yan, D. H.: Distributed model of hydrological cycle system in Heihe River basin: I. Model development and Verification, J. Hydraul. Eng., 5, 003, 2006
Jiang, Z., Shen, Y., Ma, T., Zhai, P., and Fang, S.: Changes of precipitation intensity spectra in different regions of mainland China during 1961–2006, J. Meteorol. Res., 28, 1085–1098, 2014.
Kisi, O.: An innovative method for trend analysis of monthly pan evaporations, J. Hydrol., 527, 1123–1129, 2015.
Li, Y., Chen, X., and Wang, Z.: A Tentative Discussion on the Impact of Human Activities on the Variability of Runoff Serises of the Beijiang River Basin, J. Nat. Res., 21, 12–29, 2006.
Li, Y., Huang, Q., Xu, J., and Zhang, Z.: Long-range Correlations Diagnoses and Trend Prediction of RunoffOver Yellow RiverDuring the Resent 80 Years, J. Arid Land Resour. Environ., 22, 68–72, 2008.
Lin, K., He, Y., and Chen, X.: Identifying the quantitative effect of climate change and human activity on runoff in the Dongjiang River basin, J. Hydraul. Eng., 43, 1312–1321, 2012.
Liu, C. and Cheng, L.: Analysis on Runoff Series with Special Reference to Drying up Courses of Lower Huanghe River, Acta Geogr. Sin., 55, 257–265, 2000.
Mathlouthi, M. and Lebdi, F.: Event in the case of a single reservoir: the Ghèzala dam in Northern Tunisia, Stoch. Environ. Res. Risk Asses., 22, 513–528, 2008.
McVicar, T. R., Roderick, M. L., Donohue, R. J., Li, L. T., Van Niel, T. G., Thomas, A., Grieser, J., Jhajharia, D., Himri, Y., Mahowald, N. M., and Mescherskaya, A. V.: Global review and synthesis of trends in observed terrestrial near-surface wind speeds: Implications for evaporation, J. Hydrol., 416–417, 182–205, 2012.
Pettit, N. E., Froend, R. H., and Davies, P. M.: Identifying the natural flow regime and the relationship with riparian vegetation for two contrasting western Australian rivers, Regul. River., 17, 201–215, 2001.
Petts, G.: Complex response of river channel morphology subsequent to reservoir construction, Prog. Phys. Geogr., 3, 329–362, 1979.
Petts, G.: Long-term consequences of upstream impoundment, Environ. Manage., 7, 325–332, 1980.
Poff, N. L., Allan, J. D., Bain, M. B., Karr, J. R., Prestegaard, K. L., Richter, B. D., Sparks, R. E., and Stromberg, J. C.: The Natural Flow Regime, BioScience, 47, 769–784, 1997.
Prakash, S., Gairola, R. M., and Mitra, A. K.: Comparison of large-scale global land precipitation from multisatellite and reanalysis products with gauge-based GPCC data sets, Theor. Appl. Climatol., 121, 303–317, 2015.
Ren, L., Zhang, W., Li, C., and Wang, M.: Impacts of Human Activities on River Runoff in North China, J. Hohai Univ., 29, 13–18, 2001.
Richter, B. D., Baumgartner, J. V., Powell, J., and Braun, D. P.: A Method for Assessing Hydrologic Alteration within Ecosystems, Conserv. Biol., 10, 1163–1174, 1996.
Richter, B., Baumgartner, J., Wigington, R., and Braun, D.: How much water does a river need?, Freshw. Biol. 37, 231–249, 1997.
Richter, B. D., Baumgartner, J. V., Braun, D. P., and Powell, J.: A spatial assessment of hydrologic alteration within a river network, Reg. Rivers Res. Manage., 14, 329–340, 1998.
Shiau, J. T. and Wu, F. C.: Assessment of hydrologic alterations caused by Chi-Chi diversion weir in Chou-Shui Creek, Taiwan: opportunities for restoring natural flow conditions, Regul. River., 20, 401–412, 2004.
Sun, J. and Ao, J.: Changes in precipitation and extreme precipitation in a warming environment in China, Chinese Sci. Bull., 58, 1395–1401, 2013.
Szilagyi, J.: Modeled areal evaporation trends over the conterminous United States, J. Irrig. Drain. Eng., 4, 196–200, 2001.
Thame, R. A.: Global Perspective on Environmental Flow Assessment: Emerging Trends in the Development and Application of Environmental Flow Methodologies, River Res. Appl., 19, 397–441, 2013.
Wen, G., Huang, G., Tao, W., and Liu, C.: Observed trends in light precipitation events over global land during 1961–2010, Theor. Appl. Climatol., 125, 1–13, 2015.
Zhang, N., He, H. M., Zhang, S. F., Jiang, X. H., Xia, Z. Q., and Huang, F.: Influence of Reservoir Operation in the Upper Reaches of the Yangtze River (China) on the Inflow and Outflow Regime of the TGR-based on the Improved SWAT Model, Water Resour. Manage., 26, 691–705, 2012.